Documentation Revision Date: 2018-07-13
Data Set Version: 1
Summary
Multiple approaches were used to estimate the carbon fluxes. Statistical models were used for riverine input of total organic carbon (TOC), estuarine NEP, estuarine burial, and the exchange of CO2 between shelf waters and the atmosphere. Mechanistic models were employed to quantify shelf water NEP and the riverine input of dissolved inorganic carbon (DIC). Simple averaging of the available data in the study domain was used to estimate NPP in all ecosystems, tidal wetland burial and lateral export, and the exchange of CO2 between estuarine waters and the atmosphere. The remaining fluxes were computed by difference assuming steady-state balances of inorganic carbon, organic carbon, or total carbon.
There is one data file with this dataset in comma-separated format (.csv).
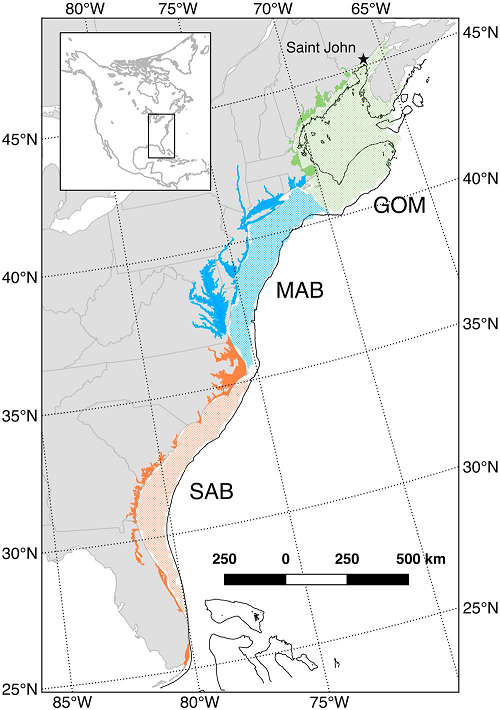
Figure 1. Study domain indicating locations of estuaries (darker colors) and shelf waters (lighter shading) in the three subregions: the Gulf of Maine (GOM), the Mid-Atlantic Bight (MAB), and the South Atlantic Bight (SAB). The 100-m isobath is shown as a solid black line. The outline of the St. John estuary in Canada was not available, so it is indicated by a star.
Citation
Najjar, R.G., M. Herrmann, R. Alexander, E.W. Boyer, D.J. Burdige, D. Butman, W. Cai, E.A. Canuel, B. Chen, M. Friedrichs, R.A. Feagin, P. Griffith, A. Hinson, J.R. Holmquist, X. Hu, W.M. Kemp, K. Kroeger, A. Mannino, S.L. Mccallister, W.R. Mcgillis, M. Mulholland, C. Pilskaln, J. Salisbury, S. Signorini, P. St-laurent, H. Tian, M.A. Tzortziou, P. Vlahos, Z. Wang, and R. Zimmerman. 2018. Mean Annual Fluxes of Carbon in Coastal Ecosystems of Eastern North America. ORNL DAAC, Oak Ridge, Tennessee, USA. https://doi.org/10.3334/ORNLDAAC/1594
Table of Contents
- Data Set Overview
- Data Characteristics
- Application and Derivation
- Quality Assessment
- Data Acquisition, Materials, and Methods
- Data Access
- References
Data Set Overview
This dataset contains best estimates and uncertainties for mean annual fluxes of inorganic, organic, and total (organic + inorganic) carbon in tidal wetlands, estuaries and shelf waters of eastern North America, which is defined by the coastline running between the tip of the Scotian Peninsula (Canada) and the southern tip of Florida (USA). The data are provided on a per-unit-area basis and as spatially integrated values for each of the three ecosystems types (tidal wetlands, estuaries, and shelf waters) and the entire coastal ecosystem (tidal wetlands + estuaries + shelf waters) as well as for three geographic subregions (the Gulf of Maine, the Mid-Atlantic Bight, and the South Atlantic Bight) and the entire Eastern North America domain (Gulf of Maine + Mid-Atlantic Bight + South Atlantic Bight). The data include the net uptake from the atmosphere by the three ecosystems; burial in tidal wetland soils, estuarine sediments, and continental shelf sediments; riverine input from land to estuaries; and the net lateral advective transports from ecosystem to ecosystem. In addition, heterotrophic respiration (HR), net primary production (NPP), and net ecosystem production (NEP) estimates were computed for each ecosystem. The fluxes were derived using a variety of sources and are estimates for average conditions over the past decades from data covering roughly the period 1976-01-01 to 2017-12-31.
Multiple approaches were used to estimate the carbon fluxes. Statistical models were used for riverine input of TOC, estuarine NEP, estuarine burial, and the exchange of CO2 between shelf waters and the atmosphere. Mechanistic models were employed to quantify shelf water NEP and the riverine input of dissolved inorganic carbon (DIC). Simple averaging of the available data in the study domain was used to estimate NPP in all ecosystems, tidal wetland burial and lateral export, and the exchange of CO2 between estuarine waters and the atmosphere. The remaining fluxes were computed by difference assuming steady-state balances of inorganic carbon, organic carbon, or total carbon.
Related Publication
Najjar, R.G., M. Herrmann, R. Alexander, E. W. Boyer, D. J. Burdige, D. Butman, W.-J. Cai, E. A. Canuel, R. F. Chen, M. A. M. Friedrichs, R. A. Feagin, P. C. Griffith, A. L. Hinson, J. R. Holmquist, X. Hu, W. M. Kemp, K. D. Kroeger, A. Mannino, S. L. McCallister, W. R. McGillis, M. R. Mulholland, C. H. Pilskaln , J. Salisbury, S. R. Signorini, P. St-Laurent , H. Tian, M. Tzortziou, P. Vlahos, Z. A. Wang, and R. C. Zimmerman. 2018. Carbon budget of tidal wetlands, estuaries, and shelf waters of eastern North America. Global Biogeochemical Cycles, 32, 389–416. https://doi.org/10.1002/2017GB005790
Acknowledgements
The dataset was generated with support from the following programs: NASA NACP, grant number NNX14AM37G; NASA IDS, grant number NNX14AF93G; NASA Ocean Biology and Biogeochemistry Program, grant number NNX11AD47G; NSF Chemical Oceanography Program, grant number OCE-1260574
Data Characteristics
Spatial Coverage: Eastern North America, which is defined as the eastern coastal areas of the US and Canada, from the tip of the Scotian Peninsula (Canada) to the southern tip of Florida (USA)
Spatial Resolution: Flux per square meter within an ecosystem and as spatially integrated values for each of the three ecosystems
Temporal Coverage: The estimates were derived from data covering the approximate period from 1976-01-01 to 2017-12-31
Temporal Resolution: The data are annual estimates for average conditions over the past decades
Study Area (coordinates in decimal degrees)
Site | Westernmost Longitude | Easternmost Longitude | Northernmost Latitude | Southernmost Latitude |
---|---|---|---|---|
Eastern coastal areas of the USA and Canada | -85E | -65E | 45N | 25N |
Data File Information
This dataset contains one data file in comma-separated format (east_coast_carbon_fluxes.csv). The file provides the mean carbon fluxes with uncertainties (reprted as two standard errors of the mean) on a per-unit-area basis and as spatially integrated values for each of the three ecosystems as well as for three geographic subregions.
Important User Note: For consistency across ecosystem domains, the convention of a flux from the atmosphere as a positive number was adopted, even though atmospheric scientists and oceanographers often use the opposite sign convention.
Table 1. Variables in the data file, east_coast_carbon_fluxes.csv
Variable name | Units/format | Description |
---|---|---|
ecotype | Ecosystem type: 1W: tidal wetlands 2E: estuaries (including seagrasses) 3S: shelf waters 4C: entire coastal domain (sum of all ecotypes) |
|
fluxtype | Flux types: refer to Table 2 below for codes and definitions | |
region | Geographic region: GOM: Gulf of Maine MAB : Mid-Atlantic Bight SAB: South Atlantic Bight ENA: Eastern North America (sum of GOM, MAB, and SAB) |
|
flux_unit_area | g C m-2 yr-1 | Mean carbon flux per unit area of regional ecotype (exept for NPPSG, which is per unit area of seagrass). See User Note above. |
flux_unit_area_uncertainty | g C m-2 yr-1 | Uncertainty in carbon flux per unit area reported as two standard errors of the mean |
flux_integral | Tg C yr-1 | Mean carbon flux spatially integrated over regional ecotype. See User Note above. |
flux_integral_uncertainty | Tg C yr-1 | Uncertainty in spatially integrated carbon flux reported as two standard errors of the mean |
Table 2. Flux-type descriptions
Fluxtype | Description |
---|---|
B | Burial (loss of organic carbon) |
LES_I | Lateral transport from estuaries to shelf of inorganic carbon |
LES_O | Lateral transport from estuaries to shelf of organic carbon |
LES_T | Lateral transport from estuaries to shelf of total carbon |
LSO_I | Lateral transport from shelf to open ocean of inorganic carbon (negative values indicates net transport in the opposite direction, from ocean to shelf) |
LSO_O | Lateral transport from shelf to open ocean of organic carbon (negative values indicate net transport in the opposite direction, from ocean to shelf) |
LSO_T | Lateral transport from shelf to open ocean of total carbon (negative values indicate net transport in the opposite direction, from ocean to shelf) |
LW_I | Total lateral transport out of wetlands of inorganic carbon |
LW_O | Total lateral transport out of wetlands of organic carbon |
LW_T | Total lateral transport out of wetlands of total carbon |
LWE_I | Lateral transport from wetlands to estuaries of inorganic carbon |
LWE_O | Lateral transport from wetlands to estuaries of organic carbon |
LWE_T | Lateral transport from wetlands to estuaries of total carbon |
LWS_I | Lateral transport from wetlands to shelf of inorganic carbon |
LWS_O | Lateral transport from wetlands to shelf of organic carbon |
LWS_T | Lateral transport from wetlands to shelf of total carbon |
NEP | Net ecosystem production (source of organic carbon, loss of inorganic carbon; NEP=NPP-HR; negative values indicate net heterotropy) |
R_I | Inputs from rivers to estuaries of inorganic carbon |
R_O | Inputs from rivers to estuaries of organic carbon |
R_T | Inputs from rivers to estuaries of total carbon (organic+inorganic) |
U | Net uptake from the atmosphere (source of inorganic carbon; negative values indicate net outgassing to the atmosphere) |
D | Degassing by tidal wetlands (NPPW - UW) |
HR | Heterotrophic respiration (loss of organic carbon, source of inorganic carbon) |
NPP | Net primary production (source of organic carbon, loss of inorganic carbon) |
NPPSG | Seagrass NPP (included in estuarine NPP; per unit area of seagrass) |
Application and Derivation
An understanding of carbon cycling in the coastal zone has benefits that extend beyond the global carbon cycle. Defining and balancing the carbon budget takes on some urgency due to rapid coastal development, accelerating climate change, and the need to better quantify the role of coastal ecosystems as sources and sinks of carbon. This study shows that coastal carbon budgets should explicitly consider tidal wetlands, estuaries, shelf waters, and the linkages between them; ignoring any of these components may produce a biased picture of coastal carbon cycling.
Quality Assessment
Data uncertainties are provided in the data file. Errors in flux per unit area were estimated using a variety of approaches. When possible, formal error estimates were used from data-constrained models. Fluxes based on data syntheses were assigned standard errors by taking the standard deviation of the mean. Inter-annual variability was used as a proxy for error for some model-based fluxes. Finally, when error estimates were not possible using quantitative approaches, expert judgment was used.
Data Acquisition, Materials, and Methods
Study Domain
The study domain (Figure 1) extends from the southern tip of the Scotian Peninsula (Canada) to the southern tip of Florida (USA). The domain was divided into three subregions, which are, from north to south, the Gulf of Maine (GOM), the Mid-Atlantic Bight (MAB), and the South Atlantic Bight (SAB).
The subregions differ considerably in their physical characteristics. About a quarter of the watershed of Eastern North America drains to the GOM, and the remainder drains approximately evenly to the MAB and SAB. Nevertheless, runoff to each subregion is about the same, reflecting regional differences in precipitation and evapotranspiration.
Table 3. Geographic area of each ecotype (in units of 103km2)
Region |
Tidal wetlands (uncertainty as two standard errors) |
Estuaries | Shelf waters | Entire coastal domain |
---|---|---|---|---|
Gulf of Maine (GOM) | 0.51 (0.27) |
5.69 | 186.00 | 192.20 |
Mid-Atlantic Bight (MAB) | 3.61 (0.72) |
20.30 | 93.00 | 116.91 |
South Atlantic Bight (SAB) | 6.01 (0.92) |
12.18 | 102.00 | 120.19 |
Eastern North America (ENA) | 10.13 (1.20) |
38.18 | 381.00 | 429.30 |
Methods and Data Sources
The carbon flux data were obtained from a variety of sources including historical data, empirical models, remote sensing algorithms, and process-based models to determine the carbon fluxes for the three coastal ecosystems (tidal wetlands, estuaries, and shelf waters). A table summarizing the data sources is provided at the end of this section (Table 4). U.S. estuarine surface area was taken from Herrmann et al. (2015), whose primary sources were the National Oceanic and Atmospheric Administration’s National Estuarine Eutrophication Assessment survey (Bricker et al., 2007) and a digital spatial framework based on a U.S. Geological Survey watershed hierarchy known as the Coastal Assessment Framework (NOAA, 1985).
Constructing individual carbon budgets for all Canadian GOM estuaries was not possible as there was no readily available estuarine inventory for the Canadian portion of the GOM. However, there was information concerning carbon fluxes (Clair et al., 2013) and characteristics of the St. John River, which accounts for most of the streamflow entering the GOM at the Canadian coastline; thus, the only Canadian estuary explicitly included in our analysis is the St. John River estuary.
U.S. tidal wetland areas were taken from Hinson et al. (2017), who distributed tidal wetland areas from the U.S. National Wetlands Inventory (Dahl & Stedman, 2013) among estuarine and coastal drainage areas. ENA tidal wetlands are mostly emergent vegetation (73%) and freshwater tidal (21%), with the remainder (6%) being shrub-scrub and forested. Wetlands that drain to estuaries were distinguished from wetlands that drain directly to shelf waters. In the U.S. portion of the study area, 14.5% of the tidal wetland area drains directly to shelf waters. Canadian GOM tidal wetland area was taken from Hanson and Calkins (1996). Lacking knowledge of the location of Canadian tidal wetlands, it was assumed that they all drain directly to the ocean.
Flux Estimations
A variety of approaches were used to estimate the carbon fluxes given the heterogeneity of the coastal zone and the scarcity of observations. Statistical models were used that were strongly constrained by observations in the study domain. Such was the case for riverine input of TOC (Shih et al., 2010), estuarine NEP and burial (Herrmann et al., 2015), and the exchange of CO2 between shelf waters and the atmosphere (Signorini et al., 2013). Mechanistic models were employed to quantify shelf water NEP (St-Laurent et al., 2017) and the riverine input of dissolved inorganic carbon (DIC) (Tian et al., 2015). Where regional, data constrained models did not exist, simple averaging of the available data in the study domain was used. This approach was applied for NPP in all ecosystems, tidal wetland burial and lateral export, and the exchange of CO2 between estuarine waters and the atmosphere. When regional models and data were not available, generic models were used, as in the case of burial in shelf sediments (Burdige, 2007). Finally, numerous fluxes were computed by difference. Total carbon fluxes were computed as sums of organic and inorganic carbon fluxes, except for exchange between shelf and open ocean waters, in which case the total carbon balance was used. In addition, heterotrophic respiration (HR), net primary production (NPP), and net ecosystem production (NEP) estimates were computed for each ecosystem. The net lateral transports of TOC, DIC, and total carbon from shelf waters to the open ocean were computed as residuals.
Errors in flux per unit area were estimated using a variety of approaches. When possible, formal error estimates were used from data-constrained models. Fluxes based on data syntheses were assigned standard errors by taking the standard deviation of the mean. Inter-annual variability was used as a proxy for error for some model-based fluxes. Finally, when error estimates were not possible using quantitative approaches, expert judgment was used.
The flux estimates are briefly described below.
Tidal Wetland Fluxes
Net Primary Production of Tidal Wetlands (NPPW)
The computed subregional averages and standard errors were used from wetland aboveground (or aerial) NPP and belowground NPP measurements in the United States (Continental Shelf Associates Inc. (1991, p. 91), and Mendelssohn and Morris (2000)). The standard errors are based on 132 aboveground NPP estimates (18, 76, and 38 respectively from the GOM, MAB, and SAB) and 45 belowground NPP estimates (5, 29, and 11 respectively from the GOM, MAB, and SAB).
Burial in Tidal Wetlands (BW)
Estimates of tidal wetland burial in the study region are based on the global synthesis of carbon accumulation rate by Ouyang and Lee (2014), which contains 59 estimates in the study region (28, 23, and 8, respectively, from the GOM, MAB, and SAB). Carbon accumulation rate is the product of carbon density and sediment accumulation rate.
Net Lateral Flux from Tidal Wetlands (LWE and LWS)
The net lateral flux of total organic carbon (TOC = particulate organic carbon + dissolved organic carbon = POC + DOC) and DIC was estimated from tidal wetlands to estuaries and shelf waters. The net lateral DIC flux was computed from tidal wetlands in ENA (Morris & Whiting, 1986; Neubauer & Anderson, 2003; Wang et al., 2016; Wang & Cai, 2004). The computed means and standard errors of the net lateral TOC and DIC fluxes were applied to all ENA tidal wetlands, independent of subregion.
Tidal Wetland Fluxes Computed as Residuals (NEPW and UW)
NEPW and UW were computed as residuals in the carbon budget assuming steady state.
Estuarine Fluxes
Estuarine Net Primary Production (NPPE)
Estuarine NPP is assumed to be the sum of NPP from plankton and seagrass. Estimates of estuarine planktonic primary production were taken from the global synthesis of Cloern et al. (2014), which lists median annual production for 24 ENA estuaries (3, 15, and 6 from the GOM, MAB, and SAB, respectively), based on a total of 159 individual annual production estimates for specific sites and years.
Seagrass
The approach of Hill et al. (2014) was used to estimate seagrass production in situ, in which NPP is the product of standing carbon biomass (standing = aboveground + belowground) and an assumed specific growth rate.
Estuarine Net Ecosystem Production (NEPE)
Estuarine net ecosystem production (NEPE) for U.S. East Coast estuaries estimates by Herrmann et al. (2015), were used and modified by (1) incorporating the new TOC fluxes from tidal wetlands and (2) include the Canadian portion of the GOM.
Riverine Input (R)
The Spatially Referenced Regression on Watersheds attributes (SPARROW) model was used to provide estimates of riverine TOC fluxes to tidal estuarine waters. SPARROW is a spatially explicit statistical model that exploits data on land use, flow, and constituent concentration.
Exchange of CO2 Between Estuaries and the Atmosphere (UE)
For the exchange of CO2 Between Estuaries and the Atmosphere (UE), 16 literature estimates of UE were compiled (Cai & Wang, 1998; Crosswell et al., 2014, 2012; Hunt et al., 2014, 2011; Jiang et al., 2008; Joesoef et al., 2015; Raymond & Hopkinson, 2003; Raymond et al., 2000; Wang & Cai, 2004), and means and standard errors were computed for each subregion.
Estuarine Burial (BE)
Estimates of carbon burial in estuarine sediments of U.S. East Coast estuaries were taken from the model of Herrmann et al. (2015), which requires riverine total nitrogen loading and estuarine residence time as inputs. The model was applied to the St. John River estuary using the residence time of Metcalfe et al. (1976) and the riverine total nitrogen loading of Clair et al. (2013). To estimate burial in all Canadian GOM estuaries, the St. John estimate was multiplied by the freshwater scaling factor of 1.69.
Estuarine Fluxes Computed as Residuals (LOES and LIES)
As in Herrmann et al. (2015), LOES was determined from the estuarine organic balance. Similarly, the estuarine inorganic carbon balance was used to calculate LIES.
Shelf Water Fluxes
Shelf Water Net Primary Production (NPPS)
Syntheses of in situ shelf water net primary production measurements were derived from two major programs. The first is a set of National Marine Fisheries Service surveys from October 1977 to June 1982 in the MAB and GOM. More than 4,000 measurements were made with good spatial and seasonal coverage (O’Reilly & Busch, 1984; O’Reilly et al., 1987). The second major source is the Department of Energy’s interdisciplinary study of the oceanography of the SAB. Menzel (1993) summarized the NPP measurements from this program.
Shelf Water Net Ecosystem Production (NEPS)
Estimates of shelf water net ecosystem production (NEPS) were taken from the latest version (St-Laurent et al., 2017) of the process-based numerical model developed by the U.S. Eastern Continental Shelf (USECoS) project.
Exchange of CO2 Between Shelf Waters and the Atmosphere (US)
Estimates of the exchange of CO2 between ENA shelf waters and the atmosphere were taken from Signorini et al. (2013), who used the Ho et al. (2011) gas transfer velocity and a satellite-based pCO2 algorithm for the period 2003–2010 in all three subregions. Signorini et al. (2013) treated Georges Bank and Nantucket Shoals as a separate region; for this study, however, these were combined with the GOM.
Burial in Shelf Sediments
Shelf organic carbon burial fluxes were estimated by starting with a model of sedimentary organic carbon oxidation.
Shelf Water Fluxes Computed as Residuals (LSO)
The net lateral transports of TOC, DIC, and total carbon from shelf waters to the open ocean were computed as residuals. Refer to Najjar et al. (2018) for additional details.
Table 4. Summary of data sources, computed or otherwise, cited in this documentation from the Najjar et al. (2018) study.
Source Data | Reference |
---|---|
US estuarine surface area | Herrmann et al. (2015); Bricker et al. (2007); NOAA (1985) |
Canadian GOM estuaries-carbon fluxes and characteristics of the St. John River | Clair et al. 2013 |
U.S. tidal wetland areas | Hinson et al. (2017); Dahl & Stedman (2013) |
Canadian GOM tidal wetland area | Hanson and Calkins (1996) |
Riverine input of TOC | Shih et al. (2010) |
Estuarine NEP and burial | Herrmann et al. (2015) |
Exchange of CO2 between shelf waters and the atmosphere | Signorini et al. (2013) |
Shelf water NEP | St-Laurent et al. (2017) |
Riverine input of dissolved inorganic carbon (DIC) | Tian et al. (2015) |
Burial in shelf sediments (modeled data) | Burdige, 2007 |
Net lateral transports of TOC, DIC, and total carbon from shelf waters to the open ocean | Najjar et al. (2018) |
Subregional averages and standard errors were used from wetland aboveground (or aerial) NPP and belowground NPP measurements in the United States | Continental Shelf Associates Inc. (1991, p. 91), and Mendelssohn and Morris (2000) |
Estimates of tidal wetland burial in the study region | Ouyang and Lee (2014) |
Tidal Wetland Fluxes Computed as Residuals (NEPW and UW) | Computed data (Najarra et al. (2018)) |
Estuarine planktonic primary production | Cloern et al. (2014) |
Seagrass production in situ | Hill et al. (2014) |
Estuarine net ecosystem production (NEPE) for U.S. East Coast estuaries. These estimates were modified. | Herrmann et al. (2015) |
Riverine TOC fluxes to tidal estuarine waters | SPAtially Referenced Regression on Watersheds attributes (SPARROW) |
Exchange of CO2 Between Estuaries and the Atmosphere | Cai & Wang (1998); Crosswell et al. (2014, 2012); Hunt et al. (2014, 2011); Jiang et al. (2008); Joesoef et al. (2015); Raymond & Hopkinson (2003); Raymond et al. (2000); Wang & Cai (2004) |
Carbon burial in estuarine sediments of U.S. East Coast estuaries (modeled data) and the St. John River estuary | Herrmann et al. (2015), Metcalfe et al. (1976), Clair et al. (2013) |
Estuarine fluxes computed as residuals | Computed from the estuarine organic balance |
In situ shelf water net primary production measurements | National Marine Fisheries Service surveys from October 1977 to June 1982, (O’Reilly & Busch (1984); O’Reilly et al. (1987)); the Department of Energy’s interdisciplinary study of the oceanography of the SAB summarized by Menzel (1993) |
Shelf water net ecosystem production | St-Laurent et al. (2017) |
The exchange of CO2 between ENA shelf waters and the atmosphere | Signorini et al. (2013); Ho et al. (2011) used for the gas transfer velocity |
Shelf organic carbon burial fluxes | Modeled data ((Najjar et al. (2018)) |
Net lateral transports of TOC, DIC, and total carbon from shelf waters to the open ocean | Computed as residuals ((Najjar et al. (2018)) |
Net Lateral Flux from Tidal Wetlands (LWE and LWS) | Morris & Whiting (1986); Neubauer & Anderson (2003); Wang et al. (2016); Wang & Cai (2004) |
Data Access
These data are available through the Oak Ridge National Laboratory (ORNL) Distributed Active Archive Center (DAAC).
Mean Annual Fluxes of Carbon in Coastal Ecosystems of Eastern North America
Contact for Data Center Access Information:
- E-mail: uso@daac.ornl.gov
- Telephone: +1 (865) 241-3952
References
Bricker, S., Longstaff, B., Dennison, W., Jones, A., Boicourt, K., Wicks, C., & Woerner, J. (2007). Effects of Nutrient Enrichment in the Nation’s Estuaries: A Decade of Change, NOAA Coastal Ocean ProgramDecision Analysis Series (Vol. 26, p. 328). Silver Spring, MD: National Centers for Coastal Ocean Science.
Burdige, D. J. (2007). Preservation of organic matter in marine sediments: Controls, mechanisms, and an imbalance in sediment organic carbon budgets? Chemical Reviews, 107(2), 467–485. https://doi.org/10.1021/cr050347q
Cai, W.-J., & Wang, Y. (1998). The chemistry, fluxes, and sources of carbon dioxide in the estuarine waters of the Satilla and Altamaha Rivers, Georgia. Limnology and Oceanography, 43(4), 657–668. https://doi.org/10.4319/lo.1998.43.4.0657
Clair, T. A., Dennis, I. F., & Bélanger, S. (2013). Riverine nitrogen and carbon exports from the Canadian landmass to estuaries. Biogeochemistry, 115(1-3), 195–211. https://doi.org/10.1007/s10533-013-9828-2
Cloern, J. E., Foster, S. Q., & Kleckner, A. E. (2014). Phytoplankton primary production in the world’s estuarine-coastal ecosystems. Biogeosciences, 11(9), 2477–2501. https://doi.org/10.5194/bg-11-2477-2014
Continental Shelf Associates Inc. (1991). A comparison of marine productivity among outer continental shelf planning areas. Supplement-An evaluation of benthic habitat primary productivity. A final report for the U.S. Department of the Interior, Minerals Management Service, Herndon, VA. OCS study MMS 91-0001. Contract No. 14-35-0001-30487. 245 pp. + app.
Crosswell, J. R., Wetz, M. S., Hales, B., & Paerl, H.W. (2012). Air-water CO2 fluxes in the microtidal Neuse River estuary, North Carolina. Journal of Geophysical Research, 117, C08017. https://doi.org/10.1029/2012JC007925
Crosswell, J. R., Wetz, M. S., Hales, B., & Paerl, H. W. (2014). Extensive CO2 emissions from shallow coastal waters during passage of Hurricane Irene (August 2011) over the mid-Atlantic Coast of the USA. Limnology and Oceanography, 59(5), 1651–1665. https://doi.org/10.4319/lo.2014.59.5.1651
Dahl, T. E., & Stedman, S. M. (2013). Status and trends of wetlands in the coastal watersheds of the conterminous United States 2004 to 2009 (p. 46). Washington, DC: U.S. Department of the Interior, Fish and Wildlife Service and National Oceanic and Atmospheric Administration, National Marine Fisheries Service.
Hanson, A., & Calkins, L. (1996). Wetlands of the Maritime Provinces: Revised documentation for the wetlands inventory (Tech. Rep. 267, 67). Atlantic Region, Sackville, New Brunswick: Environment Canada, Canadian Wildlife Service.
Herrmann, M., Najjar, R. G., Kemp, W. M., Alexander, R. B., Boyer, E. W., Cai, W.-J., et al. (2015). Net ecosystem production and organic carbon balance of U.S. East Coast estuaries: A synthesis approach. Global Biogeochemical Cycles, 29, 96–111. https://doi.org/10.1002/2013GB004736
Hill, V. J., Zimmerman, R. C., Bissett, W. P., Dierssen, H., & Kohler, D. D. (2014). Evaluating light availability, seagrass biomass, and productivity using hyperspectral airborne remote sensing in Saint Joseph’s Bay, Florida. Estuaries and Coasts, 37(6), 1467–1489. https://doi.org/10.1007/s12237-013-9764-3
Hinson, A. L., Feagin, R. A., Eriksson, M., Najjar, R. G., Herrmann, M., Bianchi, T. S., et al. (2017). The spatial distribution of soil organic carbon in tidal wetland soils of the continental United States. Global Change Biology, 23(12), 5468–5480. https://doi.org/10.1111/gcb.13811
Ho, D. T., Wanninkhof, R., Schlosser, P., Ullman, D. S., Hebert, D., & Sullivan, K. F. (2011). Toward a universal relationship between wind speed and gas exchange: Gas transfer velocities measured with 3He/SF6 during the Southern Ocean Gas Exchange Experiment. Journal of Geophysical Research, 116, C00F04. https://doi.org/10.1029/2010JC006854
Hunt, C. W., Salisbury, J. E., & Vandemark, D. (2014). CO2 input dynamics and air–sea exchange in a large New England estuary. Estuaries and Coasts, 37(5), 1078–1091. https://doi.org/10.1007/s12237-013-9749-2
Hunt, C. W., Salisbury, J. E., Vandemark, D., & McGillis, W. (2011). Contrasting carbon dioxide inputs and exchange in three adjacent New England estuaries. Estuaries and Coasts, 34(1), 68–77. https://doi.org/10.1007/s12237-010-9299-9
Jiang, L.-Q., Cai, W.-J., & Wang, Y. (2008). A comparative study of carbon dioxide degassing in river- and marine-dominated estuaries. Limnology and Oceanography, 53(6), 2603–2615. https://doi.org/10.4319/lo.2008.53.6.2603
Joesoef, A., Huang, W.-J., Gao, Y., & Cai, W.-J. (2015). Air–water fluxes and sources of carbon dioxide in the Delaware estuary: Spatial and seasonal variability. Biogeosciences, 12(20), 6085–6101. https://doi.org/10.5194/bg-12-6085-2015
Mendelssohn, I. A., & Morris, J. T. (2000). Eco-physiological controls on the productivity of Spartina Alterniflora Loisel. In M. P. Weinstein & D. A. Kreeger (Eds.), Concepts and Controversies in Tidal Marsh Ecology (pp. 59–80). Netherlands: Springer.
Menzel, D. W. (1993). Ocean processes: US Southeast continental shelf. A summary of research conducted in the South Atlantic Bight under the auspices of the US Department of Energy From 1977 to 1991 (p. 112). Savannah: US Dept. of Energy, Office of Scientific and Technical Information.
Metcalfe, C. D., Dadswell, M. J., Gillis, G. F., & Thomas, M. L. H. (1976). Physical, chemical, and biological parameters of the Saint John River Estuary (Tech. Rep. 686, 41 pp.), New Brunswick, Canada: Fisheries and Marine Service, Environment Canada.
Morris, J. T., & Whiting, G. J. (1986). Emission of gaseous carbon dioxide from salt-marsh sediments and its relation to other carbon losses. Estuaries and Coasts, 9(1), 9–19. https://doi.org/10.2307/1352188
Najjar, R.G., M. Herrmann, R. Alexander, E. W. Boyer, D. J. Burdige, D. Butman, W.-J. Cai, E. A. Canuel, R. F. Chen, M. A. M. Friedrichs, R. A. Feagin, P. C. Griffith, A. L. Hinson, J. R. Holmquist, X. Hu, W. M. Kemp, K. D. Kroeger, A. Mannino, S. L. McCallister, W. R. McGillis, M. R. Mulholland, C. H. Pilskaln , J. Salisbury, S. R. Signorini, P. St-Laurent , H. Tian, M. Tzortziou, P. Vlahos, Z. A. Wang, and R. C. Zimmerman. (2018). Carbon budget of tidal wetlands, estuaries, and shelf waters of eastern North America. Global Biogeochemical Cycles, 32, 389–416. https://doi.org/10.1002/2017GB005790
Neubauer, S. C., & Anderson, I. C. (2003). Transport of dissolved inorganic carbon from a tidal freshwater marsh to the York River estuary. Limnology and Oceanography, 48(1), 299–307. https://doi.org/10.4319/lo.2003.48.1.0299
NOAA (1975). The coastline of the United States, NOAA/PA 71046.
NOAA (1985). National estuarine inventory data atlas. Volume 1: Physical and hydrologic characteristics. Washington, DC: National Ocean Service, National Oceanic and Atmospheric Administration.
O’Reilly, J., & Busch, D. A. (1984). Phytoplankton primary production on the northwestern Atlantic shelf, rapports et Proces-verbaux des Réunions. Conseil International pour l’Éxploration de la Mer, 183, 255–268.
O’Reilly, J., Evans-Zetlin, C., & Busch, D. A. (1987). Primary production. In R. H. Backus (Ed.), Georges bank (pp. 220–233). Cambridge, MA: MIT Press.
Ouyang, X., & Lee, S. (2014). Updated estimates of carbon accumulation rates in coastal marsh sediments. Biogeosciences, 11(18), 5057–5071.https://doi.org/10.5194/bg-11-5057-2014
Raymond, P. A., Bauer, J. E., & Cole, J. J. (2000). Atmospheric CO2 evasion, dissolved inorganic carbon production, and net heterotrophy in the York River estuary. Limnology and Oceanography, 45(8), 1707–1717. https://doi.org/10.4319/lo.2000.45.8.1707
Raymond, P. A., & Hopkinson, C. S. (2003). Ecosystem modulation of dissolved carbon age in a temperate marsh-dominated estuary. Ecosystems, 6(7), 694–705. https://doi.org/10.1007/s10021-002-0213-6
Shih, J.-S., Alexander, R. B., Smith, R. A., Boyer, E. W., Schwarz, G. E., & Chung, S. (2010). An initial SPARROW model of land use and in-stream controls on total organic carbon in streams of the conterminous United States, U.S. Geol. Surv. Open File Rep. 2010–1276, 22 pp., Reston, VA.
Signorini, S., Mannino, A., Friedrichs, M. A. M., Najjar, R. G., Cai, W.-J., Salisbury, J. E., et al. (2013). Surface ocean pCO2 seasonality and sea-air CO2 flux estimates for the North American east coast. Journal of Geophysical Research: Oceans, 118, 5439–5460. https://doi.org/10.1002/jgrc.20369
St-Laurent, P., Friedrichs, M. A. M., Najjar, R. G., Herrmann, M., Miller, S., Martins, D., & Wilkin, J. (2017). Impacts of atmospheric nitrogen deposition on surface waters of the western North Atlantic mitigated by multiple feedbacks. Journal of Geophysical Research: Oceans, 122, 8406–8426. https://doi.org/10.1002/2017JC013072
Tian, H. Q., Yang, Q. C., Najjar, R. G., Ren, W., Friedrichs, M. A. M., Hopkinson, C. S., & Pan, S. (2015). Anthropogenic and climatic influences on carbon fluxes from eastern North America to the Atlantic Ocean: A process-based modeling study. Journal of Geophysical Research: Biogeosciences, 120, 757–772. https://doi.org/10.1002/2014JG002760
Wang, Z. A., Kroeger, K. D., Ganju, N. K., Gonneea, M. E., & Chu, S. N. (2016). Intertidal salt marshes as an important source of inorganic carbon to the coastal ocean. Limnology and Oceanography, 61(5), 1916–1931. https://doi.org/10.1002/lno.10347
Wang, Z. A., & Cai,W. J. (2004). Carbon dioxide degassing and inorganic carbon export from a marsh-dominated estuary (the Duplin River): A marsh CO2 pump. Limnology and Oceanography, 49(2), 341–354. https://doi.org/10.4319/lo.2004.49.2.0341