Documentation Revision Date: 2024-10-18
Dataset Version: 1
Summary
Marsh soil formation rates and chemical properties are influenced by physical and biogeochemical processes that vary from site to site. Chen and Twilley (1999) developed the NUMAN model to predict soil accretion rates and chemical properties in mangrove environments. Twilley et al. (2024) adapted NUMAN for marsh environments to create the NUMAR. NUMAR requires precise input parameters to produce reliable results.
This dataset includes one compressed file (.zip) which contains a shapefile and two files in comma-separated values (.csv) format.
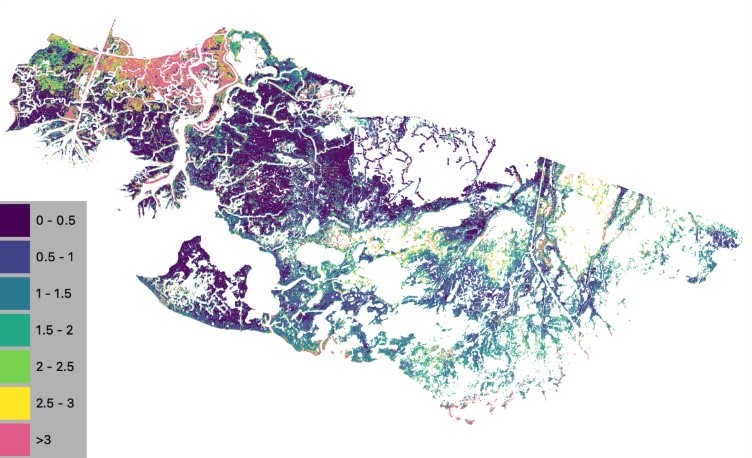
Figure 1. Mean accretion rate (cm per year) in Atchafalaya and Terrebonne basins in coastal Louisiana over a 79 year simulation from 2021-2100.
Citation
Twilley, R., P. Biswas, A. Rovai, A.L. Christensen, A.F. Cassaway, and I.A. Vargas-Lopez. 2024. Delta-X: NUMAR Soil Accretion Modeled to 2100, MRD, Louisiana, USA. ORNL DAAC, Oak Ridge, Tennessee, USA. https://doi.org/10.3334/ORNLDAAC/2368
Table of Contents
- Dataset Overview
- Data Characteristics
- Application and Derivation
- Quality Assessment
- Data Acquisition, Materials, and Methods
- Data Access
- References
Dataset Overview
This dataset holds modeled estimates of soil accretion for the Atchafalaya and Terrebonne basins in the Mississippi River Delta of coastal Louisiana, U.S. Soil accretion was predicted from 2021-2100 using the Numerical Understanding of Marsh Accretion Resilience (NUMAR) model. Marsh soil formation rates and chemical properties are influenced by physical and biogeochemical processes that vary from site to site. Chen and Twilley (1999) developed the NUMAN model to predict soil accretion rates and chemical properties in mangrove environments. Twilley et al. (2024) adapted NUMAN for marsh environments to create the NUMAR. NUMAR is process-based and requires precise input parameters to produce reliable results. The input parameters were aggregated within ecogeomorphic cells, areas of similar vegetation and elevation. The dataset includes spatially explicit input values, description of important parameters, and a shapefile of model outputs.
Project: Delta-X
The Delta-X mission is a 5-year NASA Earth Venture Suborbital-3 mission to study the Mississippi River Delta in the United States, which is growing and sinking in different areas. River deltas and their wetlands are drowning as a result of sea level rise and reduced sediment inputs. The Delta-X mission will determine which parts will survive and continue to grow, and which parts will be lost. Delta-X begins with airborne and in situ data acquisition and carries through data analysis, model integration, and validation to predict the extent and spatial patterns of future deltaic land loss or gain.
Related Datasets
Twilley, R., P. Biswas, A. Rovai, A.F. Cassaway, and I.A. Vargas-lopez. 2024. Delta-X: NUMAR Predictive Model for Marsh Accretion Rates and Chemical Properties. ORNL DAAC, Oak Ridge, Tennessee, USA. https://doi.org/10.3334/ORNLDAAC/2354
- Provides input data and model code to run the NUMAR model
Castañeda-Moya, E., and E. Solohin. 2023. Delta-X: Soil Properties for Herbaceous Wetlands, MRD, Louisiana, 2021, V3. ORNL DAAC, Oak Ridge, Tennessee, USA. https://doi.org/10.3334/ORNLDAAC/2239
- Provides properties of soil core samples for herbaceous wetlands collected in the Atchafalaya and Terrebonne basins
Castañeda-Moya, E., and E. Solohin. 2022. Delta-X: Belowground Biomass and Necromass across Wetlands, MRD, LA, USA, 2021, V2. ORNL DAAC, Oak Ridge, Tennessee, USA. https://doi.org/10.3334/ORNLDAAC/2238
Castañeda-Moya, E., and E. Solohin. 2023. Delta-X: Aboveground Biomass and Necromass across Wetlands, MRD, Louisiana, 2021, V2. ORNL DAAC, Oak Ridge, Tennessee, USA. https://doi.org/10.3334/ORNLDAAC/2237
- Provides in situ measurements of root biomass.
Cortese, L., and S. Fagherazzi. 2023. Delta-X: Delft3D Broad-Scale Sediment Model, Terrebonne Basin, MRD, Louisiana, USA. ORNL DAAC, Oak Ridge, Tennessee, USA. https://doi.org/10.3334/ORNLDAAC/2301
- Provides simulated water levels, velocity, and depth-averaged sediment concentrations in the Atchafalaya and Terrebonne basins
Jensen, D.J., E. Castañeda-Moya, E. Solohin, A. Rovai, D.R. Thompson, and M. Simard. 2023. Delta-X: AVIRIS-NG L3 Derived Aboveground Biomass, MRD, Louisiana, USA, 2021, V2. ORNL DAAC, Oak Ridge, Tennessee, USA. https://doi.org/10.3334/ORNLDAAC/2138
- Provides gridded estimates of herbaceous aboveground biomass (AGB) for the Atchafalaya and Terrebonne basins
Jensen, D.J., E. Castañeda-Moya, E. Solohin, D.R. Thompson, and M. Simard. 2024. Delta-X AVIRIS-NG L3 Derived Vegetation Types, MRD, Louisiana, USA. ORNL DAAC, Oak Ridge, Tennessee, USA. https://doi.org/10.3334/ORNLDAAC/2352
- Provides vegetation type classification across the Atchafalaya and Terrebonne basins
Simard, M., M.W. Denbina, E. Rodriguez, A.L. Christensen, K.A. Wright, and A. Rovai. 2022. Delta-X: Ecogeomorphic Cell Products across the MRD, LA, USA, 2021. ORNL DAAC, Oak Ridge, Tennessee, USA. https://doi.org/10.3334/ORNLDAAC/2108
- This product delineates the Mississippi River Delta (MRD) landscape into distinct ecogeomorphic cells
Acknowledgement:
This program was funded under the NASA Earth Venture Suborbital-3 Program, grant number NNH17ZDA001N-EVS3.
Data Characteristics
Spatial Coverage: Atchafalaya and Terrebonne Basins, southern coast of Louisiana, USA
Temporal Coverage: 2021-01-01 to 2100-12-31
Temporal Resolution: One-time estimate after 79 years of simulation
Site Boundaries: Latitude and longitude are given in decimal degrees.
Site | Westernmost Longitude | Easternmost Longitude | Northernmost Latitude | Southernmost Latitude |
Atchafalaya and Terrebonne Basins | -91.540 | -90.411 | 29.700 | 29.079 |
Data File Information
This dataset includes one compressed file (.zip) which contains a shapefile and two files in comma-separated values (.csv) format.:
DeltaX_NUMAR_Landscape_results_shapefile.zip: Simulation results in shapefile; multiple files in a compressed zip archive. Polygon data are in geographic coordinates (EPSG 4326). Polygon attributes are listed in Table 1.
DeltaX_NUMAR_Landscape_Inputs.csv: Spatially explicit model inputs (Table 2).
DeltaX_NUMAR_parameters_by_class: Parameter values used to populate the ecogeomorphic cells (Table 3).
Table 1. Polygon attributes in the file Delta-X_NUMAR_Landscape_results_shapefile.zip
Variable | Attribute label | Units | Description |
---|---|---|---|
site_id | site_id | - | Unique identifier of the ecogeomorphic cell |
Total Accretion (cm) | TA_79yrs | cm | Total accretion for a soil column built during a defined simulation period |
Mean Accretion (cm/yr) | SAR | cm y-1 | Soil accretion per year |
Mean OM% | OMpercent | percent | Mean organic matter concentration (% dry mass) in the soil formed during simulation period |
Mean OM Concentration (mg/cm3) | OMD | mg cm-3 | Mean organic matter density in soil formed during simulation period |
Mean BD | BD | g cm-3 | Mean bulk density in soil formed during simulation period |
C_Seq | CSeq | g m-2 y-1 | Mean carbon sequestration in soil formed during simulation period |
OC Concentration | OCD | mg cm-3 | Mean organic carbon density in soil formed during simulation period |
IM | IMpercent | percent | Mean inorganic matter percentage in soil formed during simulation period |
IM Concentration | IMD | mg cm-3 | Mean inorganic matter density in soil formed during simulation period |
Necromass | Tnec | Mg ha-1 | Estimated total necromass in soil formed during simulation period |
Root Necromass | RTnec_tot | Mg ha-1 | Estimated total root necromass in soil formed during simulation period |
Root Necromass Projected Up to 50 cm | RTnec_50c | Mg ha-1 | Estimated total root necromass projected up to 50 cm soil depth |
Table 2. Variables in the file DeltaX_NUMAR_Landscape_Inputs.csv
Variables | Units | Description | Source |
---|---|---|---|
label | - | Site_id. Unique identifier of the ecogeomorphic cell | |
basin | - | Basin (active or inactive) | Modified from HUC 8 polygons |
vegtype | - | Vegetation type | Delta-X L3 Derived Vegetation Type modified using USGS/CPRA Vegetation Type (Jensen et al., 2024; CPRA, 2024) |
agb | Mg h-1 | Aboveground biomass | Delta-X L3 Derived Aboveground Biomass (Jensen et al., 2023) |
imar | g cm-2 y-1 | Loaded inorganic matter per year | Delta-X Campaign (Cortese and Fagherazzi, 2022; Cortese and Fagherazzi, 2023) |
oms | g cm-2 y-1 | Organic matter deposition rate | Cassaway et al. (2024) |
si | g cm-2 y-1 | Inorganic matter deposition rate (same as imar, renamed for the model run) | Delta-X Delft3d Sediment Model (Cortese et al., 2023; Cortese and Fagherazzi, 2023) |
r0 | g cm-2 y-1 | Root biomass at the surface | Derived using root:shoot measured in field (Castañeda-Moya and Solohin, 2022; Castañeda-Moya and Solohin, 2023a) and aboveground biomass (Jensen et al., 2023) |
id | - | Indexed cell | |
geometry | - | Defined boundary of the ecogeomorphic cell; allows for import into GIS software | Delta-X Ecogeomorphic Cells (Simard et al., 2022) |
Note: For variables b0, bi, c0, c1, c2, c4, fc1, kb, kc, kl, kr, and e, see Table 3.
Table 3. Variables in the file DeltaX_NUMAR_parameters_by_class.csv
Variable | Units | Description | Source |
---|---|---|---|
Classname | - | Classification of the vegetation | CPRA-CRMS shapefiles (Nyman et al., 2023) and from Delta-X campaign (Jensen et al., 2024) |
Class | - | Indexed the vegetation type | Cassaway et al. (2024); Twilley et al. (2023) |
bo | g cm-3 | Self-packing density of organic matter | Estimated from coastwide CPRA-CRMS soil core measurements for the purpose of landscape coastal application (CPRA, 2024) |
bi | g cm-3 | Self-packing density of inorganic matter | |
c0 | g g-1 | Lignin content in the surface deposit | Fontenot (2022) |
c1 | g g-1 | Ash content in the root biomass | |
c2 | g g-1 | Cellulose content in the surface deposit | Considered negligible; no data available |
c4 | g g-1 | Cellulose content in the root biomass | Wilson (1985) |
fc1 | g g-1 | Lignin content in the root biomass | Fontenot (2022) |
kb | y-1 | Belowground decomposition rate of labile organic matter | |
kc | y-1 | Cellulose decomposition rate | Means et al. (1985) |
kl | y-1 | Lignin decomposition rate | |
kr | y-1 | Root turnover rate | Estimated from Twilley et al. (2024) |
e | cm-1 | Root attenuation rate | Determined from Castañeda-Moya and Solohin (2023) |
bgbagb | 1 | Ratio of belowground biomass to aboveground biomass | From experimental belowground to aboveground ratio for six sites (Castañeda-Moya and Solohin, 2022; Castañeda-Moya and Solohin, 2023a) |
omssi | 1 | Ratio of organic matter deposition to inorganic matter deposition rate; measured over feldspar in feldspar plots | Cassaway et al. (2024) |
Application and Derivation
Chen and Twilley (1999) developed the NUMAN model to predict soil accretion rates and chemical properties in mangrove environments. Twilley et al. (2024) adapted NUMAN for marsh environments to create the NUMAR model for these simulations.
These model results illustrate spatial variation in soil accretion rates that maintain and create marsh habitats in the Atchafalaya and Terrebonne basins of the Mississippi River Delta. This user guide describes the operational workflow used for these simulations.
Quality Assessment
None provided.
Data Acquisition, Materials, and Methods
Marsh soil formation rates and chemical properties are influenced by physical and biogeochemical processes that vary from site to site. To predict soil accretion rates and chemical properties in mangrove environments, Chen and Twilley (1999) developed the NUMAN model. The Numerical Understanding of Marsh Accretion Resilience (NUMAR) was created to adapt NUMAN for marsh environments (Twilley et al., 2024). NUMAR is process-based and requires precise input parameters to predict the soil accretion and other soil properties across a marsh landscape.
This document provides a guideline on how to adapt the NUMAR Unit model for a landscape-level simulation. It describes how to prepare the input table with required parameters to initialize the NUMAR Landscape simulations. The NUMAR Unit model is provided separately as a set of Python scripts (Twilley et al., 2024). The input variables required to initialize the NUMAR Unit model are included in this dataset.
NUMAR Landscape Simulations Operational Workflow
The NUMAR Unit model can be applied to a specific site, multiple sites, or across gridded surface landscapes to produce maps of long-term (i.e., multi-decadal) sediment accretion. For whichever purpose, NUMAR Unit model requires a suite of input parameters to be initialized. There are essentially two main ways to populate the NUMAR input table, from a lookup reference values table (e.g., values obtained from field observations or compiled from the literature) and/or from geospatial products (e.g., remotely sensed-based maps or modeled outputs from either vector or raster files). To run NUMAR Unit model for a specific or fewer sites, required variables can be entered manually in the input table. To run NUMAR Unit model over larger gridded surface landscapes (which may be referred to as a NUMAR Landscape simulations), a template raster for a desired coastal region extent (i.e., model domain) can be used where each raster degree cell represents individual ‘sites’ containing a unique pair of latitude and longitude coordinates for cells’ centroids that can be used to extract values from other ancillary vector and raster files containing required input data to initialize NUMAR Unit model.
An example is provided of a NUMAR Landscape simulations where sets of values used to initialize NUMAR Unit model were derived from both geospatial products (raster and vector) and a lookup table containing site-specific reference values for sites along a transect ranging from freshwater to brackish to saline marshes in coastal Louisiana. These site-specific values were obtained during multiple Delta-X mission field sampling campaigns between October 2019 and October 2023.
NUMAR Landscape Simulations - Simulated surface sediment deposition rates
To apply the NUMAR model across the Delta-X landscape, input parameters were produced across the Atchafalaya and Terrebonne domain. The parameters were aggregated within ecogeomorphic cells (Simard et al., 2022), areas of similar vegetation and elevation determined using a segmentation algorithm to cluster pixels with similar elevation and optical properties. For more information on these cells, users should reference the user guide for Simard et al. (2022) (https://doi.org/10.3334/ORNLDAAC/2108).
Each ecogeomorphic cell was assigned to a class: active fresh, active brackish, active saline, inactive fresh, inactive brackish, inactive saline based on available geospatial data. Active and inactive basins were designated using modified Hydrologic Unit Codes (HUC) basins for the Atchafalaya and Terrebonne basins. The modification included transferring the areas around Fourleague Bay to the Atchafalaya basin because of the influence of Atchafalaya River sediment to the bay (Figure 2).
Figure 2. Hydrologic basins used to determine active and inactive cells.
The salinity zone of each ecogeomorphic cell was determined by using the Delta-X L3 derived vegetation type product (Jensen et al., 2024) and the USGS/CPRA vegetation type product (Nyman et al., 2023). Modifications to the Delta-X L3 vegetation type product included adding a brackish type, which includes any Saltmarsh Grass pixels that fall within the Brackish zone in the USGS/CPRA product. In addition, any Saltmarsh Grass pixels that fall within the Fresh or Swamp zone in the USGS/CPRA product are re-classified as Freshwater Grass. Figure 3 shows the modified vegetation type product. More information about the input products can be found in the user guide for Jensen et al. (2024) (https://doi.org/10.3334/ORNLDAAC/2352) and documentation for Nyman et al. (2023) (https://doi.org/10.5066/P9URYLMS).
Figure 3. Vegetation type modified from Delta-X L3 Derived Vegetation Type from Jensen et al. (2023) using data from Nyman et al. (2023).
The final classes assigned to each ecogeomorphic cell (Figure 3) were used to determine specific input parameters based on site-specific field measurements. For more information, users should reference the user guide for the NUMAR model (Twilley et al., 2024) (https://doi.org/10.3334/ORNLDAAC/2354).
Figure 4. Ecogeomorphic classes based on vegetation type and basin type.
Aboveground biomass for herbaceous and forested vegetation were derived from the Delta-X L3 product (Jensen et al., 2023). The extent of aboveground biomass is limited to the flight path of AVIRIS-NG. Spring and fall aboveground biomass data were averaged within each ecogeomorphic cell (Figure 4). For more information, consult the user guide for Jensen et al. (2023) (https://doi.org/10.3334/ORNLDAAC/2138).
Figure 5. Aboveground biomass derived from AVIRIS-NG products by Jensen et al. (2024).
Belowground biomass was calculated using site-specific field measurements of belowground biomass to aboveground biomass ratios and root attenuation rates. These were applied using a lookup table.
Mineral sediment deposition rates (Figure 6) for the Atchafalaya and Terrebonne basins were derived from the Delta-X Delft3D Broad-Scale Sediment Models (Cortese et al., 2023). These models simulate hydrodynamics, waves, and sediment transport on a 90-m spatial resolution and provide annual inorganic mass accumulation rates (IMAR) in g cm-2 yr-1. IMAR data were averaged within each ecogeomorphic cell. For more information on these model products, users should reference the user guides for Cortese et al., (2023) (https://doi.org/10.3334/ORNLDAAC/2302 and Cortese and Fagherazzi (2023)(https://doi.org/10.3334/ORNLDAAC/2301).
Figure 6. Mineral sediment deposition derived from Delft3D sediment transport models by Cortese et al. (2023).
Organic matter deposition rates were calculated by multiplying IMAR by the ratio of organic matter to inorganic matter loading rate. This ratio comes from site-specific field measurements and are applied using a lookup table.
All remaining input parameters were assigned using a lookup table based on the classes discussed above.
Data Access
These data are available through the Oak Ridge National Laboratory (ORNL) Distributed Active Archive Center (DAAC).
Delta-X: NUMAR Soil Accretion Modeled to 2100, MRD, Louisiana, USA
Contact for Data Center Access Information:
- E-mail: uso@daac.ornl.gov
- Telephone: +1 (865) 241-3952
References
Cassaway, A. F., R.R. Twilley, A.S. Rovai, and G.A. Snedden. 2024. Patterns of marsh surface accretion rates along salinity and hydroperiod gradients between active and inactive coastal deltaic floodplains. Estuarine, Coastal and Shelf Science 301:108757. https://doi.org/10.1016/j.ecss.2024.108757
Castañeda-Moya, E., and E. Solohin. 2022. Delta-X: Belowground Biomass and Necromass across Wetlands, MRD, LA, USA, 2021, V2. ORNL DAAC, Oak Ridge, Tennessee, USA. https://doi.org/10.3334/ORNLDAAC/2238
Castañeda-Moya, E., and E. Solohin. 2023a. Delta-X: Aboveground Biomass and Necromass across Wetlands, MRD, Louisiana, 2021, V2. ORNL DAAC, Oak Ridge, Tennessee, USA. https://doi.org/10.3334/ORNLDAAC/2237
Castañeda-Moya, E., and E. Solohin. 2023b. Delta-X: Soil Properties for Herbaceous Wetlands, MRD, Louisiana, 2021, V3. ORNL DAAC, Oak Ridge, Tennessee, USA. https://doi.org/10.3334/ORNLDAAC/2239
Chen, R., and R.R. Twilley. 1999. A simulation model of organic matter and nutrient accumulation in mangrove wetland soils. Biogeochemistry 44:93–118. https://doi.org/10.1007/BF00993000
CPRA. 2024. Coastwide Reference Monitoring System-Wetlands Monitoring Data. Coastal Information Management System (CIMS) database. Coastal Protection and Restoration Authority (CPRA) of Louisiana. Accessed 25 June 2024. https://cims.coastal.louisiana.gov
Cortese, L., and S. Fagherazzi. 2022. Fetch and distance from the bay control accretion and erosion patterns in Terrebonne marshes (Louisiana, USA). Earth Surface Processes and Landforms 47:1455–1465. https://doi.org/10.1002/esp.5327
Cortese, L., and S. Fagherazzi. 2023. Delta-X: Delft3D Broad-Scale Sediment Model, Terrebonne Basin, MRD, Louisiana, USA. ORNL DAAC, Oak Ridge, Tennessee, USA. https://doi.org/10.3334/ORNLDAAC/2301
Cortese, L., X. Zhang, and S. Fagherazzi. 2023. Delta-X: Delft3D Broad-Scale Sediment Model, Atchafalaya Basin, MRD, Louisiana, USA. ORNL DAAC, Oak Ridge, Tennessee, USA. https://doi.org/10.3334/ORNLDAAC/2302
Fontenot, A. 2022. Wetland Soil Development Along Salinity and Hydrogeomorphic Gradients in Active and Inactive Deltaic Basins of Coastal Louisiana. [Master of Science, Louisiana State University and Agricultural and Mechanical College]. https://doi.org/10.31390/gradschool_theses.5622
Jensen, D.J., E. Castañeda-Moya, E. Solohin, A. Rovai, D.R. Thompson, and M. Simard. 2023. Delta-X: AVIRIS-NG L3 Derived Aboveground Biomass, MRD, Louisiana, USA, 2021, V2. ORNL DAAC, Oak Ridge, Tennessee, USA. https://doi.org/10.3334/ORNLDAAC/2138
Jensen, D.J., E. Castañeda-Moya, E. Solohin, D.R. Thompson, and M. Simard. 2024. Delta-X AVIRIS-NG L3 Derived Vegetation Types, MRD, Louisiana, USA. ORNL DAAC, Oak Ridge, Tennessee, USA. https://doi.org/10.3334/ORNLDAAC/2352
Means, J.E., K. Cromack Jr, and P.C. MacMillan. 1985. Comparison of decomposition models using wood density of Douglas-fir logs. Canadian Journal of Forest Research 15:1092–1098. https://doi.org/10.1139/x85-178
Nyman, J.A., C.S. Reid, C.E. Sasser, J. Linscombe, S.B. Hartley, B.R. Couvillion, and R.K. Villani. 2023. Vegetation Types in Coastal Louisiana in 2021 (ver. 2.0, April 2023). U.S. Geological Survey. https://doi.org/10.5066/P9URYLMS
Simard, M., M.W. Denbina, E. Rodriguez, A.L. Christensen, K.A. Wright, and A. Rovai. 2022. Delta-X: Ecogeomorphic Cell Products across the MRD, LA, USA, 2021. ORNL DAAC, Oak Ridge, Tennessee, USA. https://doi.org/10.3334/ORNLDAAC/2108
Twilley, R., P. Biswas, A. Rovai, A.F. Cassaway, and I.A. Vargas-lopez. 2024. Delta-X: NUMAR Predictive Model for Marsh Accretion Rates and Chemical Properties. ORNL DAAC, Oak Ridge, Tennessee, USA. https://doi.org/10.3334/ORNLDAAC/2354
Wilson, J. O. 1985. Decomposition of [14C]Lignocelluloses of Spartina alterniflora and a comparison with field experiments. Applied and Environmental Microbiology 49:478–484. https://doi.org/10.1128/aem.49.3.478-484.1985