Documentation Revision Date: 2015-10-06
Data Set Version: V1
Summary
This project was funded by NASA’s Interdisciplinary Science (IDS) and Carbon Monitoring System (CMS) programs.
These data were used in combination with an atmospheric transport model to derive total average CH4 emissions from the urban region, the fractional contribution of natural gas to total methane emissions, and the loss rate of natural gas to the atmosphere.
There are eight comma-separated (*.csv) data files with this data set.
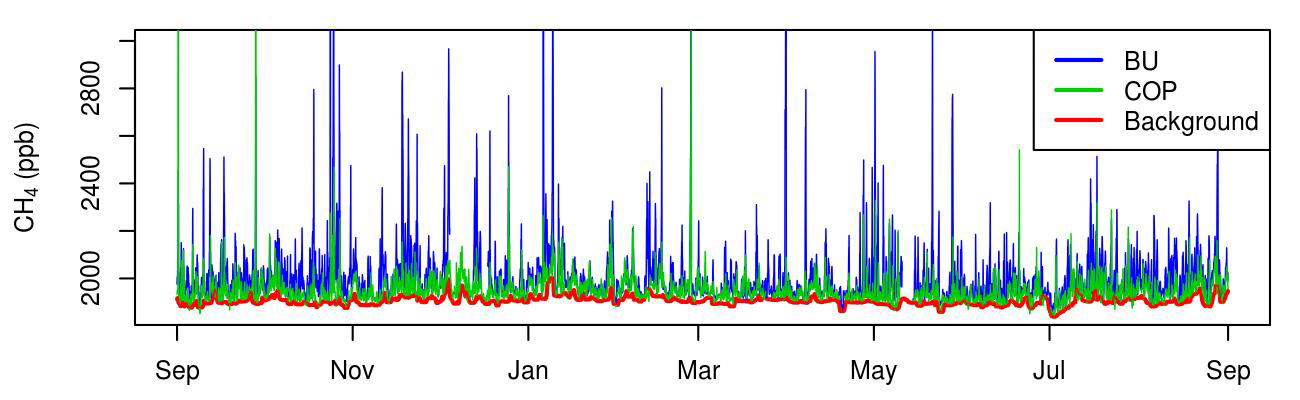
Figure 1. Mean hourly measured CH4 concentrations at two urban sites (BU and COP) and in the empirical background from September 1, 2012 through August 31, 2013
Citation
McKain, K., A. Down, S.M. Raciti, J.W. Budney, L.R. Hutyra, C. Floerchinger, S.C. Herndon, T. Nehrkorn, M.S. Zahniser, R.B. Jackson, N. Phillips, and S.C. Wofsy. 2015. CMS: Atmospheric Methane Concentrations and Prior Emissions, Boston, MA, 2012-2014. ORNL DAAC, Oak Ridge, Tennessee, USA. http://dx.doi.org/10.3334/ORNLDAAC/1291 .
Table of Contents
- Data Set Overview
- Data Characteristics
- Application and Derivation
- Quality Assessment
- Acquisition Materials and Methods
- Data Access
- References
Data Set Overview
Users are requested to reference the data citation (above) and also to reference the following publication when these data are used:
McKain,K., A. Down, S.M. Racitie, J. Budney, L.R. Hutyra, C. Floerchinger, S.C. Herndon, T.Nehrkorn, M.S. Zahniser, R.B. Jackson, N. Phillips, and S.C. Wofsy. Methane emissions from natural gas infrastructure and use in the urban region of Boston, Massachusetts. PNAS. 112:1941-1946. DOI: 10.1073/pnas.1416261112.
Atmospheric CH4 concentrations were measured continuously from September 2012 through August 2013 at four locations: Boston University (BU), Copley Square (COP), Harvard Forest (HF) and Nahant (NHT). Ethane concentrations were measured with a laser spectrometer (Yacovitch et al., 2014) at BU for three months in the fall and winter of 2012–2013 and one month in the late spring of 2014.
Background concentrations in air flowing into the city were estimated by randomly sampling from a range (5th to 35th) of lower percentiles of CH4 measurements from two upwind stations (HF or NHT, depending on the direction of simulated air trajectories), averaged over a 48-h moving window.
Methane enhancements were modeled at BU and COP with the Stochastic Time-Inverted Lagrangian Transport (STILT) model (Lin et al., 2003) coupled to the Weather Research and Forecasting (WRF) meso-scale meteorological model run at 1-km2 grid resolution (Nehrkorn et al., 2010). A spatially resolved prior model of CH4 emissions was constructed for the study region and combined with WRF-STILT footprints to generate a set of simulated CH4 enhancement values for each hour at each measurement station. The emission inventory was scaled to estimate optimized CH4 emission rates for the region by season and as an annual average.
Project: Carbon Monitoring System (CMS)
The CMS is designed to make significant contributions in characterizing, quantifying, understanding, and predicting the evolution of global carbon sources and sinks through improved monitoring of carbon stocks and fluxes. The System will use the full range of NASA satellite observations and modeling/analysis capabilities to establish the accuracy, quantitative uncertainties, and utility of products for supporting national and international policy, regulatory, and management activities. CMS will maintain a global emphasis while providing finer scale regional information, utilizing space-based and surface-based data.
Data Characteristics
Spatial Coverage
An ~18,000 km^2 area centered on Boston, Massachusetts.
Spatial Resolution
- Methane and ethane measurements were collected at the respective sites.
-
Methane fluxes and NG consumption were estimated for 1-km^2 grid cells in a 90-km radius circle around Boston.
Temporal Coverage
Data are for the periods September-December 2012, January-August 2013, and May-June, 2014.
Temporal Resolution
- Methane measurements are given as hourly averages. Ethane data are given as five-minute medians.
- Prior CH4 fluxes and NG consumption are given as temporally invariant.
Study Area (All latitudes and longitudes are given in decimal degrees.)
Site | Westernmost Longitude | Easternmost Longitude | Northernmost Latitude | Southernmost Latitude | Ground Elevation above Sea Level (m) | Sampling Height above Ground (m) |
---|---|---|---|---|---|---|
Boston University (BU), Boston, Massachusetts | -71.104 | -71.104 | 42.350 | 42.350 | 4 | 29 |
Copley Square (COP), Boston, Massachusetts | -71.084 | -71.084 | 42.347 | 42.347 | 7 | 215 |
Harvard Forest (HF), Massachusetts | -72.171 | -72.171 | 42.538 | 42.538 | 340 | 29 |
Nahant (NHT), Massachusetts | -70.909 | -70.909 | 42.424 | 42.424 | 15 | 16 |
Data File Information
There are eight data files in comma-separated (*.csv) format with this data set:
1. BU_CH4_Obs_Mod_Bg_Sep2012-Aug2013.csv: Average hourly measured, modeled, and background methane concentrations at the Boston University (BU) site from September 2012- August 2013.
2. COP_CH4_Obs_Mod_Bg_Sep2012-Aug2013.csv: Average hourly measured, modeled, and background methane concentrations at the Copley Square (COP) site from September 2012- August 2013.
3. HF_CH4_Obs_Sep2012-Aug2013.csv: Average hourly measured methane concentrations at the Harvard Forest (HF) site from September 2012- August 2013.
4. NHT_CH4_Obs_Sep2012-Aug2013.csv: Average hourly measured methane concentrations at the Nahant (NHT) site from September 2012- August 2013.
5. BU_C2H6_CH4_5min_May-Jun2014.csv: 5-minute median measured methane and ethane concentrations at the BU site for one month in the late spring of 2014.
6. BU_C2H6_CH4_5min_Oct2012-Jan2013.csv: 5-minute median measured methane and ethane concentrations at the BU site for three months in the fall and winter of 2012-2013.
7. Prior_flux_fields.csv: Prior flux fields by sector and natural gas consumption field for study area at 1 square km spatial resolution and as temporally invariant. Prior fluxes included five anthropogenic and biogenic emission source categories: wetlands, enteric fermentation, transportation, point-sources, and natural gas losses.
8. Prior_flux_fields_Scaling_Factors.csv: Scaling factors for prior fluxes, seasonal and annual averages and 95% confidence ranges.
Data Dictionary
Note that all times are in UTC and are hour or minute centered (e.g. Hr=5 covers data collected from Hr 4.5-5.5).
Files 1 and 2: BU_CH4_Obs_Mod_Bg_Sep2012-Aug2013.csv and COP_CH4_Obs_Mod_Bg_Sep2012-Aug2013.csv
Column | Column Heading | Units/format | Description |
---|---|---|---|
1 | Yr | YYYY | Year of measurement= 2012 or 2013 |
2 | Mn | mm | Month of the year. For the year 2012, Mn=9-12 (September-December), for the year 2013, Mn= 1-8 (January-August) |
3 | Dy | dd | Day of the month in UTC |
4 | Hr | Hour of the day in UTC; data are hour-centered | |
5 | CH4_observed | ppb | Average measured atmospheric methane concentration (ppb) |
6 | CH4_Mod_enhanced | ppb | Average modeled methane concentration enhancement (ppb) |
7-37 | CH4_background_0.05-CH4_background_0.35 | ppb | Range of background methane concentration realizations, from 48-hour moving windows of 5-35 percentiles of HF and NHT measurements (ppb) |
Files 3 and 4: HF_CH4_Obs_Sep2012-Aug2013.csv and NHT_CH4_Obs_Sep2012-Aug2013.csv
Column | Column Heading | Units/format | Description |
---|---|---|---|
1 | Yr | YYYY | Year of measurement= 2012 or 2013 |
2 | Mn | mm | Month of the year. For the year 2012, Mn=9-12 (September-December), for the year 2013, Mn= 1-8 (January-August) |
3 | Dy | dd | Day of the month in UTC |
4 | Hr | Hour of the day in UTC; data are hour-centered | |
5 | CH4_observed | ppb | Average measured atmospheric methane concentration (ppb) |
Files 5 and 6: BU_C2H6_CH4_5min_May-Jun2014.csv and BU_C2H6_CH4_5min_Oct2012-Jan2013.csv
Column | Column Heading | Units/format | Description |
---|---|---|---|
1 | Yr | YYYY | Year of measurement= 2012, 2013, or 2014 |
2 | Mn | mm | Month of the year |
3 | Dy | dd | Day of the month in UTC |
4 | Hr | hh | Hour of the day in UTC |
5 | Min | ppb | Minute of the hour in UTC; data are minute-centered |
6 | CH4_observed | ppb | Median measured atmospheric methane concentration (ppb) |
7 | C2H6_observed | ppb |
Median measured atmospheric ethane concentration (ppb). |
File 7: Prior_flux_fields.csv
Column | Column Heading | Units/format | Description |
---|---|---|---|
1 | Lon | degrees | Longitude of each 1-km2 grid cell center (decimal degrees) |
2 | Lat | degrees | Latitude of each 1-km2 grid cell center (decimal degrees) |
3 | Wetland | g CH4/m2/yr | Prior fluxes from wetlands (g CH4 /m2/yr) |
4 | EntericFerm | g CH4/m2/yr | Prior fluxes from cattle enteric fermentation (g CH4/m2/yr) |
5 | Transport | g CH4/m2/yr | Prior fluxes from on-road transport (g CH4 /m2/yr) |
6 | PointSources | g CH4/m2/yr | Average emissions from the EPA Greenhouse Gas Reporting Program in 2012 and 2013 (g CH4 /m2/yr) |
7 | NatGasLoss | g CH4/m2/yr | Prior fluxes from natural gas losses (g CH4 /m2/yr) |
8 | NatGasConsump | g CH4/m2/yr | Reconstructed map of average natural gas consumption for Sept 2012 through Aug 2013 (g CH4 /m2/yr) |
File 8: Prior_flux_field_Scaling_Factors.csv
Column | Column Heading | Description |
---|---|---|
1 | Season | Season: SON=fall, DJF=winter, MAM=spring, JJA=summer, ANN=annual average |
2 | sf.mn | Average bootstrapped scaling factor |
3 | sf.lCI | Lower 95% confidence range for the bootstrapped scaling factor |
4 | sf.uCI | Upper 95% confidence range for the bootstrapped scaling factor |
Application and Derivation
These data were used in combination with an atmospheric transport model to derive total average CH4 emissions by season from the urban region, the fractional contribution of natural gas to total methane emissions, and the loss rate of natural gas to the atmosphere.
Quality Assessment
The two urban measurement sites in this study (BU and COP) were located on the tops of buildings, so special precautions were taken to ensure that the data were not contaminated with signals from very-near sources, in particular rooftop building vents.
A few small bathroom vents are located on the roof of the BU building where the measurements were made. Signals from the sewer vents on the BU roof were characterized by very short periods (1-3 s) of clearly elevated concentrations ("spikes"). These signals were eliminated from the BU CH4 data by trimming the lower and upper (to ensure no bias was imposed) 5% of the data in each hour prior to calculating hourly averages.
To avoid sampling air emitted from building vents at COP, four sample inlets were placed at the corners of the building, two stories below the top of the building, and each corner was sampled sequentially for five minutes each. The upwind corner(s) was selected as that with the lowest average concentration of CH4, CO2, or CO in each 20-minute, 4-corner sequence.
To test whether the non-continuous sampling at COP reduced the representativeness of the data, we simulated the COP sub-sampling routine with the continuous BU dataset by randomly sub- selecting one 5-minute period in each 20-minute interval. The hourly averages generated from the sub-selected data were not significantly different than hourly averages generated from the full dataset. Total data coverage for the year at the four sites was > 94%, with gaps due to maintenance, power outages, etc.
Acquisition Materials and Methods
Measurement Sites
Atmospheric CH4 concentrations were measured continuously from September 2012 through August 2013 at four locations: Boston University (BU), Copley Square (COP), Harvard Forest (HF) and Nahant (NHT). BU and COP are near the urban center and HF and NHT are outside of Boston.
- The BU measurements were made from a 2-meter tower mounted in the center of the flat rooftop of a 6-story building.
- The COP measurements were made from the 51st story of a 53-story building that stands well above surrounding structures.
- Harvard Forest measurements were made from a tower in a mixed-deciduous forest in Petersham, Massachusetts. Methane was measured sequentially at eight heights (0.3, 0.8, 4.5, 7.5, 12.7, 18.3, 24.1, and 29 m) on the tower, for four minutes each and for eight minutes at the highest (29 m) level. Methane concentrations were typically slightly depleted at the lowest measurement heights due to an oxidative soil sink. Only measurements from 29 m were used in this study.
- Nahant is a tied island northeast of Boston. Measurements were made from an unoccupied five-story building ~50 m from the coastline in a residential neighborhood, one of the "Fire Control" towers from Word War II (see http://www.coastdefense.com/nahant.htm).
Figure. 2. Location of two city (Boston University (BU), Copley Square (COP),) and two peripheral (Harvard Forest (HF); Nahant (NHT)) measurement stations (black points) in Boston, and the surrounding area. The 90-km radius circle delineates the ∼18,000- km2 land area for which CH4 emissions were calculated. The magenta and purple contours enclose 50% of the average footprint (sensitivity area) of the BU and COP afternoon measurements, respectively (McKain et al., 2015).
Measurement Methods
Methane (CH4) was measured continuously at the four sites with four different models of Picarro cavity ring down spectrometers, at intervals of 2-7 seconds, depending on the instrument model. All models measured the same spectroscopic feature of CH4. A small fraction of CH4 data points were removed when the instrument's optical cell pressure and temperature were outside 0.1 torr and 0.005 degrees C of their set-points to ensure compatibility with the instrument's spectroscopic fit parameters. Sample gas streams were not dried, so empirical H2O correction factors were used to convert measured to dry molar fractions of CH4. Instrument-specific H2O correction factors (Rella et al., 2013) were derived for all instruments except for the BU instrument, for which correction factors from the literature were applied (Rella et al., 2013). The BU instrument failed before its H2O response could be quantified in the lab.
A two-point linear calibration equation was calculated for each instrument in the field. Compressed air cylinders with known, approximately ambient CH4 concentrations were measured by each instrument for four minutes every eight hours to quantify the intercept (null value) of the calibration equation and to track long-term drift. Data from the first ~90 seconds of each standard measurement period were disregarded to ensure the surveillance measurement had equilibrated. Measured offsets were smoothed over a multi-day moving window before being applied to correct ambient CH4 measurements.
All calibration gases were manufactured by Scott-Marin (Riverside, CA) using natural air (Nara et al., 2012) and tied to scales defined by the National Oceanic and Atmospheric Administration (NOAA) and the World Meteorological Organization. Surveillance standards before and after field deployment and were all found to have changed ≤ 0.2 ppb for CH4. One surveillance tank was used at each site for the entire year of measurements.
Total analytical uncertainty in the CH4 measurements is approximated as the sum of measurement precision, uncertainty in calibration and surveillance tank values, and uncertainty in the H2O correction. Long-term drift was not included in the calculation of total analytical uncertainty because it was captured and corrected for in the data processing. Uncertainty in the H2O correction equation is estimated as ± 2 ppb at H2O concentrations up to 3.4% (Rella et al., 2013), which sufficiently captures the maximum ambient measured H2O concentration. Therefore total analytical uncertainty for one year of hourly average CH4 measurements among the four sites was ≤ ~3 ppb (95% CI), < 0.2% of ambient concentrations.
Background concentrations in air flowing into the city were estimated by randomly sampling from a range (5th to 35th) of lower percentiles of CH4 measurements from two upwind stations (HF or NHT, depending on the direction of simulated air trajectories), averaged over a 48-h moving window, to capture synoptic-scale variability and remove possible influences of small nearby sources. Values of ΔCH4 were calculated by subtracting background from urban concentrations. Hourly average ΔCH4 data were aggregated into daily afternoon (11-16 h EST, 16-21 h UTC) means to remove autocovariance and focus the analysis on periods of well-mixed atmospheric conditions.
Ethane Measurements and the Contribution of NG to Elevated CH4 Concentrations
Ethane concentrations were measured with a laser spectrometer (Yacovitch et al., 2014) at BU for three months in the fall and winter of 2012-13 and one month in the late spring of 2014. Covariances between atmospheric C2H6 and CH4 observations were determined from the daily slopes of a linear model that minimizes x2 (Shamarock and Klemp, 2008) of 5-min median afternoon data, equivalent to a Maximum Likelihood Estimation.
To quantify the fraction of the observed ΔCH4 that was due to NG emissions, we compared ratios of ethane (C2H6) and CH4 measured in the atmosphere and NG pipelines serving the region. Ethane is a significant component of NG, whereas microbial CH4 sources, such as landfills, sewage, and wetlands, produce little or no C2H6. Because Boston has no geologic CH4 seeps, no oil and gas production or refining, and low rates of biomass burning, there are no known significant sources of C2H6 in the region other than NG.
Modeled Methane Values
Methane enhancements were modeled at BU and COP with the Stochastic Time-Inverted Lagrangian Transport (STILT) model (Lin et al., 2003), coupled to the Weather Research and Forecasting (WRF) meso-scale meteorological model (Nehrkorn et al., 2010) run at 1-km2 grid resolution. WRF- STILT generates footprints (with units ΔCH4 per unit surface flux), which represent the sensitivity of each measurement point in space and time to upwind surface fluxes. Both urban measurement sites were sensitive to emissions from the greater Boston region, with COP sensitive to a larger area than BU due to its higher altitude (Figure 2).
A spatially resolved prior model of CH4 emissions was constructed for the study region and combined with WRF-STILT footprints to generate a set of simulated ΔCH4 values for each hour at each measurement station. The emission inventory was scaled for each season to equalize mean afternoon (11-16 h EST) modeled and observed ΔCH4, providing optimized CH4 emission rates for the region.
For additional information, refer to Mckain et al. (2015).
Prior Flux Fields
Natural Gas Consumption
Reports of NG consumption by state, month, and sector were obtained from the U.S. Energy Information Administration (2014) for the study area and time period. Natural gas consumption in electric power, residential, commercial, industrial, vehicle fuel, and pipeline and distribution use sectors (U.S. Energy Information Administration, 2014), were all included in the consumption estimate. Volumes of NG were converted to masses of CH4 using the ideal gas law by assuming industry standard temperature and pressure (60 degrees F and 1 atm) and 97% CH4 content, giving 1 scf NG = 1.16 moles CH4= 18.6 grams CH4.
Monthly statewide consumption by the entire electric power sector and portions of the industrial and commercial sectors (17% and 6%, respectively) was spatially allocated to individual power production facilities (U.S. Energy Information Administration, 2013-2014). Consumption by the residential and commercial sectors was spatially allocated using a parcel-level database for Massachusetts of residential and commercial building square footage and the fuel type used in each building for space and water heating (The Warren Group, Inc., 2010). This data set was constructed from multiple state and local government data sources such as Registry of Deeds, Land Court data, Town Clerk data and tax assessor information, and is widely used in the real estate industry. Vehicle fuel consumption was spatially allocated to compressed natural gas (CNG) and LNG fueling station locations (U.S. Department of Energy, 2014). Industrial sector consumption (excluding the portion accounted for by individual power production facilities) and pipeline and distribution use was spatially allocated using commercial building square footage data.
Methane Emissions
A customized emission prior with five anthropogenic and biogenic source categories and 1-km2 spatial resolution was developed for the study domain. The customized prior fluxes included five anthropogenic and biogenic emission source categories: wetlands, enteric fermentation, transportation, point-sources, and natural gas losses. The custom prior was not meant to be exhaustive, but rather was created to provide detailed emission estimates for key sectors with improved spatial resolution and accuracy.
Wetlands- Data on wetlands' location, size, and type were obtained from the National Wetlands Inventory (U.S. Fish and Wildlife Service) for the four states in the study region. An average emission rate of 7.6 g CH4 m^-2 yr^-1 was applied to the freshwater wetlands (emergent and forested/shrub) and an emission rate of 1.3 g CH4 m^-2 yr^-1 was applied to saltwater wetlands (estuarine and marine), as adopted from Bridgham et al. (2007). Areas of open water (rivers, lakes, deepwater marine) were not included in the wetlands emission layer due to a lack of emissions data from these areas. The total wetland area in the study domain of the prior was 1,900 km2, ~11% of the land area.
Enteric Fermentation- Methane emissions from ruminant livestock were spatially allocated to counties according to county-level headcounts of cattle and calves from the USDA 2007 Agricultural Census. An emission factor of 117 kg CH4 head^-1 yr^-1, the Environmental Protection Agency (EPA) emission factor for mature dairy cattle in the North Atlantic Region (U.S. Environmental Protection Agency, 1998), was multiplied by the cattle count in each county to yield a total average emission rate. Emissions from animals other than cattle were not included because cattle accounted for the majority of livestock emissions in the study area.
Transportation- Methane emissions from transportation were estimated using per-mile emission factors by vehicle type and model year (U.S. Environmental Protection Agency, 2008, 2014), state-level data on vehicle fleet composition (Federal Highway Administration, 2012), and a database of vehicle miles traveled per road (Gately et al., 2013).
Point-sources- Annual facility-level data reported to the EPA GHG Reporting Program (U.S. Environmental Protection Agency, 2014) were used to represent CH4 emissions from the largest point sources, including landfills, waste combustion, and waste water treatment plants. Data from 2012 and 2013 were weighted according to the study time period (25% in 2012 and 75% in 2013).
Natural Gas Losses- Methane emissions from NG losses were spatially allocated according to the areas of residential and commercial NG consumption and scaled so total emissions from NG in Massachusetts was equal to the state inventory estimate for 2012 (Massachusetts Executive Office of Environmental Affairs, 2014).
Data Access
This data is available through the Oak Ridge National Laboratory (ORNL) Distributed Active Archive Center (DAAC).
CMS: Atmospheric Methane Concentrations and Prior Emissions, Boston, MA, 2012-2014
Contact for Data Center Access Information:
- E-mail: uso@daac.ornl.gov
- Telephone: +1 (865) 241-3952
References
Bridgham S.D., J.P. Megonigal, J.K. Keller, N.B. Bliss, and C. Trettin (2007) Wetlands - Supplemental Materials. The First State of the Carbon Cycle Report (SOCCR): The North American Carbon Budget and Implications for the Global Carbon Cycle. A Report by the U.S. Climate Change Science Program and the Subcommittee on Global Change Research, eds AW King, et al., pp 177-192. http://cdiac.ornl.gov/SOCCR.
Federal Highway Administration (2012), Highway Statistics Series, Highway Statistics 2012, Table VM-4, www.fhwa.dot.gov/policyinformation/statistics/2012.
C.K. Gately, L.R. Hutyra, I.S. Wing, and M.N. Brondfield (2013) A bottom up approach to on-road CO2 emissions estimates: improved spatial accuracy and applications for regional planning. Env Sci Tech 47: 2423-2430.
Lin J.C., C. Gerbig, S.C. Wofsy, A.E. Andrews , B.C. Daube, K.J. Davis, and C.A. Grainger. (2003). A near-field tool or simulating the upstream influence of atmospheric observations: The Stochastic Time-Inverted Lagrangian Transport (STILT) model. J Geophys Res108: 4493-4510.
McKain, K.A., A. Down, S.M. Racitie, J. Budney, L.R. Hutyra, C. Floerchinger, S.C. Herndon, T. Nehrkorn, M.S. Zahniser, R.B. Jackson, N. Phillips, and S.C.Wofsy. Methane emissions from natural gas infrastructure and use in the urban region of Boston, Massachusetts. PNAS. February 17, 2015,vol. 112, no. 7,1941-1946. DOI 10.1073/pnas.1416261112.
Massachusetts Executive Office of Environmental Affairs (2014) Massachusetts Annual Greenhouse Gas Emissions Inventory: 1990 through 2011, with Partial 2012 Data. www.mass.gov/eea/docs/dep/air/climate/maghginv.xls.
Nara, H., H. Tanimoto, Y. Tohjima, H. Mukai, Y. Nojiri, K. Katsumata and C.W. Rella. (2012) Effect of air composition (N2, O2, Ar, and H2O) on CO2 and CH4 measurement by wavelength-scanned cavity ring-down spectroscopy: calibration and measurement strategy. AtmosMeas Tech 5: 2689-2701.
Nehrkorn T, J. Eluszkiewicz , S.C. Wofsy, J.C. Lin, C.Gerbig, M. Longo, and S. Freitas (2010) Coupled weather research and forecasting-stochastic time-inverted Lagrangian transport (WRF-STILT) model. Meteorol Atmos Phys 107:51-64.
Rella, C. W., Chen, H., Andrews, A. E., Filges, A., Gerbig, C., Hatakka, J., Karion, A., Miles, N. L., Richardson, S. J., Steinbacher, M., Sweeney, C., Wastine, B., and Zellweger, C.: High accuracy measurements of dry mole fractions of carbon dioxide and methane in humid air, Atmos. Meas. Tech., 6, 837-860, doi:10.5194/amt-6-837-2013, 2013.
Skamarock, W.C., and J.B. Klemp (2008) A time-split nonhydrostatic atmospheric model for weather research and forecasting applications. J Comput Phys 227: 3465-3485.
The Warren Group, Inc. (2010) Boston, MA, www.thewarrengroup.com.
U.S. Department of Energy (2014) Alternative Fuel Station Locator,www.afdc.energy.gov/locator/stations.
U.S. Department of Agriculture (2009), 2007 Census of Agriculture, www.agcensus.usda.gov.
U.S. Energy Information Administration (2014) Natural Gas Consumption by End Use,www.eia.gov/dnav/ng/ng_cons_sum_dcu_nus_m.htm.
U.S. Energy Information Administration (2013-2014) 2012 and 2013 Annual Electric Utility Data,Form EIA-923, www.eia.gov/electricity/data/eia923.
U.S. Environmental Protection Agency (2014) Inventory of U.S. Greenhouse Gas Emissions and Sinks: 1990-2012, EPA-430-R-14-003,www.epa.gov/climatechange/ghgemissions/usinventoryreport.html.
U.S. Environmental Protection Agency (2014) 2012 and 2013 Greenhouse Gas Emissions from Large Facilities, www.ghgdata.epa.gov/ghgp.
U.S. Environmental Protection Agency (2008) Greenhouse Gas Inventory Protocol Core Module Guidance – Direct Emissions from Mobile Combustion Sources, EPA Climate Leaders, Tables 3, A-6, and A-7.
U.S. Environmental Protection Agency (1998) AP 42, 5th edition, volume 1, Compilation of Air Pollutant Emission Factors, Chapter 14, Greenhouse Gas Biogenic Sources, Section 4, Enteric Fermentation, www.epa.gov/ttn/chief/ap42/ch14/index.html.
U.S. Fish and Wildlife Service, National Wetlands Inventory, www.fws.gov/wetlands.
U.S. National Oceanic and Atmospheric Administration, Earth System Research Laboratory, Global Monitoring Division, Central Calibration Laboratory, www.esrl.noaa.gov/gmd/ccl/ccl.html.
World Meteorological Organization (2009) 14th WMO/IAEA Meeting of Experts on Carbon Dioxide, Other Greenhouse Gases and Related Tracers Measurement Techniques WMO/TD - No. 1487 (Helsinki, Finland, 10-13 September 2007). GAW Report No. 186.
Yacovitch, T.I., S.C. Herndon, J.R. Roscioli, C. Floerchinger, R.M., McGovern, M. Agnese, G. Petron, J. Kofler, C. Sweeney, A. Karion, S.A. Conley, E.A. Kort, et al. (2014) "Demonstration of an Ethane Spectrometer for Methane Source Identification." Environmental Science & Technology. DOI: 10.1021/es501475q