Documentation Revision Date: 2024-02-20
Dataset Version: 1
Summary
There is one data file in comma-separated values (.csv) format with this dataset.
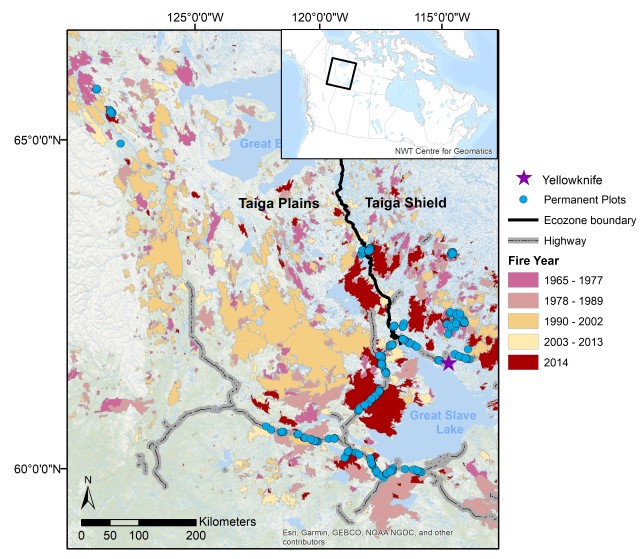
Figure 1. Locations of plots (n =511) in the Northwest Territories, Canada where soil organic layer thickness and total carbon stocks were measured. Plots were sampled along a chronosequence of time-after-fire, stratified by ecozone, and constrained by accessibility. Fire history from 1965-2014 was determined from the fire year associated with mapped fire scars (NWT Centre for Geomatics, 2012). For plots not within any recorded fire, time-after-fire was determined by tree ring analysis.
Citation
Bill, K.E., C. Dieleman, J.L. Baltzer, G.E. Degre-timmons, M.C. Mack, S.G. Cumming, X.J. Walker, and M.R. Turetsky. 2023. Post-fire Recovery of Soil Organic Layer Carbon in Canadian Boreal Forests, 2015-2018. ORNL DAAC, Oak Ridge, Tennessee, USA. https://doi.org/10.3334/ORNLDAAC/2235
Table of Contents
- Dataset Overview
- Data Characteristics
- Application and Derivation
- Quality Assessment
- Data Acquisition, Materials, and Methods
- Data Access
- References
Dataset Overview
This dataset provides site moisture, soil organic layer thickness, soil organic carbon, nonvascular plant functional group, stand dominance, ecozone, time-after-fire, jack pine proportion, and deciduous proportion for 511 forested plots spanning ~140,000 km2 across two ecozones of the Northwest Territories, Canada (NWT). The plots were established during 2015-2018 across 41 wildfire scars and unburned areas (no burn history prior to 1965), with 317 plots in the Plains and 194 plots in the Shield regions. At each plot, two adjacent 30-m transects were established 2 m apart, running north from the plot origin. Soil organic layer (SOL) depth (cm) was measured every 3 m and the mean was taken from the 10 measurements to calculate a plot-level SOL thickness. Three soil organic layer profiles were destructively sampled at 0, 12, and 24 m using a corer that was custom designed for NWT soils. Within the transects, all stems taller than 1.37 m were identified to species to calculate tree density (stems m-2). Nonvascular plant percent cover was identified to functional group at five, 1-m2 quadrats spaced 6 m apart along the belt transect. A subset of 2,067 of 5,137 total increments from 1,803 profiles from 421 plots were analyzed for total percent C using a CHN analyzer. Time-after-fire was established using fire history records. For older plots where no known fire history is recorded, tree age was used. Data are for the period 2015-06-11 to 2018-08-24 (Bill et al., 2023).
Conifer forests historically have been resilient to wildfires in part due to thick organic soil layers that regulate combustion and post-fire moisture and vegetation change. However, recent shifts in fire activity in western North America may be overwhelming these resilience mechanisms with potential impacts for energy and carbon exchange. Bill et al. (2023) quantified the long-term recovery of the organic soil layer and its carbon pools.
Project: Arctic-Boreal Vulnerability Experiment
The Arctic-Boreal Vulnerability Experiment (ABoVE) is a NASA Terrestrial Ecology Program field campaign being conducted in Alaska and western Canada, for 8 to 10 years, starting in 2015. Research for ABoVE links field-based, process-level studies with geospatial data products derived from airborne and satellite sensors, providing a foundation for improving the analysis, and modeling capabilities needed to understand and predict ecosystem responses to, and societal implications of, climate change in the Arctic and Boreal regions.
Related Datasets
There are several datasets regarding fire disturbance in the DAAC ABoVE Project collection.
Related Publication
Bill, K.,C. Dieleman, J. Baltzer, G. Degré-Timmons, M. Mack, N. Day, S. Cumming, X. Walker, and M, Turetsky. 2023. Post-fire recovery of soil organic layer carbon in Canadian boreal forests. Ecosystems (published online 2023-06-22) https://doi.org/10.1007/s10021-023-00854-0
Acknowledgement
This study was funded under the NASA ABoVE(grant NNX15AT71A) and NASA Rapid Response (grant NNX15AD58G) programs.
Data Characteristics
Spatial Coverage: Northwest Territories (NWT), Canada
ABoVE reference locations:
Domain: Core ABoVE (NWT, Canada)
Grid cells: Ah001v001, Ah002v001, Ah002v002
Temporal Resolution: One-time measurements
Temporal Coverage: 2015-06-11 - 2018-08-24
Study Area: Coordinates are provided in decimal degrees
Site | Westernmost Longitude | Easternmost Longitude | Northernmost Latitude | Southernmost Latitude |
---|---|---|---|---|
NWT | -132.6709 | -104.1943 | 68.3254 | 59.7883 |
There is one data file in comma-separated values format (.csv) with this dataset: SoilOrganicCarbonRecovery_NorthwestTerritories.csv
Table 1. Variables in the data file.
Variable | Units/format | Description |
---|---|---|
plot | - | Plot name: burn name-plot number and plot letter (e.g., "C1-14A") |
burn | - | Burn name (e.g., "C1") |
site | - | Site name: burn name-plot number (e.g., "C1-14") |
date | YYYY-MM-DD | Date of field sampling <ask DP to verify that this is the sampling date> |
decid_prop_canopy | 1 | Proportion of deciduous trees |
piba_prop_canopy | 1 | Proportion of jack pine (Pinus banksiana) |
stand_dominance | - | Common name of dominant tree species ("Jack Pine", "Black Spruce"). "Mixed" or "Deciduous" if no single species was dominant. |
time_after_fire | YY | Number of years after fire determined using fire history records. Where fire history was unknown, tree age was used. |
moisture | - | Plot moisture status: "Hydric", "Mesic", or "Xeric" |
nonvascular_functional_group | - | Functional group of nonvascular plants at plot |
avg_org_carbon | 1 | Total percent carbon determined using a CHN analyzer. Total % C was assumed to be equivalent to organic C as there is seldom inorganic C present in samples of this type |
ecozone | - | Ecozone: "plains" (Taiga Plains) or "shield" (Taiga Shield) |
avg_carbon_stock | kg m-2 | Plot-level soil organic carbon stock (kg C m-2) estimated as the mean profile C content of three soil cores and scaled to the profile surface area |
Application and Derivation
Understanding how a changing fire regime might influence these landscape and ecosystem patterns of C storage across multiple ecosystems fire cycles remains a key uncertainty, and yet it is critical for predicting the future role of the boreal biome as a net C sink or source (Walker et al., 2019). The soil organic layer (SOL) is important not only to post-fire soil C recovery but also overall vegetation stand resilience to fire (Chapin et al., 2010; Hart et al., 2018; Johnstone et al., 2016; Johnstone et al., 2010; Kasischke et al., 2010; Walker et al., 2017; Whitman et al., 2019). Bill et al. (2023) tested two main hypotheses related to SOL thickness and soil organic C stocks across an extensive plot network in the NWT.
Quality Assessment
Sampling did not always capture the entire soil organic layer in hydric plots, particularly when sampling occurred in June or July when seasonal ice was still present thereby limiting access to deeper organic soils (Bill et al., 2023).
Data Acquisition, Materials, and Methods
During 2015-2018, 511 plots were established across 41 wildfire scars and unburned areas (no burn history prior to 1965). Fire scars were selected based on fire history polygon data (NWT Centre for Geomatics, 2012) in the Northwest Territories (NWT), Canada (Figure 1). All plots were established ≥500 m from roadways, >100 m from trails, and locations without visible evidence of past resource extraction. One or two additional plots were established within a few hundred meters of the initial plot to capture local variation in soil moisture regime (Bill et al., 2023).
Site characteristics
At each plot, two adjacent 30-m transects were established 2 m apart, running north from the plot origin, form a 60-m2 area for sampling. Tree basal area (cm2 m-2) and density (stems m-2) were recorded within the plot. Diameter at breast height (DBH) was measured for stems ≥1.37 m tall. For shorter stems, basal area was measured at the base of tree.
Time-after-fire was established using fire history records. For older plots where fire history was unknown, tree age was used because most fires in boreal North America are stand replacing disturbances (Viereck et al.,1983; Greene and Johnson, 1999). To determine tree age, cores or basal cookies were taken from five trees of the (co)dominant conifer species within each plot, and their rings counted using standard dendrochronology methods to estimate age. Mean stand age was used to represent the timing of the last fire (Walker et al., 2018a).
The proportions of jack pine (Pinus banksiana), black spruce (Picea mariana), and deciduous trees (a mix of paper birch, Betula papyrifera, and trembling aspen, Populus tremuloides) were calculated using the density of stems that contributed to the canopy (>5 cm at DBH). To classify stand dominance, plots were considered to be black spruce, jack pine, or deciduous when proportion of stems of each species were greater than 50% of the stand (Walker et al., 2018a). Plots were classified as mixed where no one species was >0.5 in proportion.
Nonvascular plant percent cover was identified to functional group at five 1-m2 quadrats spaced 6 m apart along the transect. Mean percent cover was calculated by taking the mean of the five quadrats. Dominant functional group of nonvascular plants was determined using the mean nonvascular plant percent cover (>50%).
Ecozone (Taiga Plains or Taiga Shield) was derived from the Ecological Framework of Canada (Ecological Stratification Working Group, 1995).
Soil sampling and analysis
Soil organic layer (SOL) depth (cm) was measured every 3 m, and the mean was taken from 10 measurements to calculate a plot-level SOL thickness. Three SOL profiles were sampled at 0, 12, and 24 m using a corer. Soil cores captured the organic layer to either surface mineral soil or the permafrost table. All soil samples were frozen on the day of collection then shipped to University of Guelph (UoG), Ontario, Canada, or Northern Arizona University (NAU), Arizona, USA (Walker et al., 2018b) for laboratory analysis to determine bulk density and total percent carbon (%C) using a CHN analyzer.
Total %C was assumed to be equivalent to organic C (Santin et al., 2016). Relationships between soil bulk density and measured total %C were modelled to estimate SOC stocks (kg C m-2). Soil increments were classified as organic soil where total %C was greater than 20%. Samples with <20 %C were classified as mineral soil and excluded from SOC calculations. The C content of organic soil increments was calculated from the product of increment volume, bulk density and %C. Profile total SOC content was calculated by summing over the profile organic soil increments. Plot-level average SOC stocks (kg C m-2) were estimated as the mean profile C content of three soil cores, scaled to the profile surface areas.
Plots with stand age >100 years and where SOL could not be measured due to permafrost were excluded. This dataset holds records for 511 plots retained for analysis presented in Bill et al. (2023).
Data Access
These data are available through the Oak Ridge National Laboratory (ORNL) Distributed Active Archive Center (DAAC).
Post-fire Recovery of Soil Organic Layer Carbon in Canadian Boreal Forests, 2015-2018
Contact for Data Center Access Information:
- E-mail: uso@daac.ornl.gov
- Telephone: +1 (865) 241-3952
References
Bill, K.,C. Dieleman, J. Baltzer, G. Degré-Timmons, M. Mack, N. day, S. Cumming, X. Walker, and M, Turetsky. 2023. Post-fire recovery of soil organic layer carbon in Canadian boreal forests. Ecosystems (published online 2023-06-22) https://doi.org/10.1007/s10021-023-00854-0
Chapin F.S., A.D. McGuire, R.W. Ruess, T.N. Hollingsworth, M.C. Mack, J.F. Johnstone, E.S. Kasischke, J.B. Jones, M.T. Jorgenson, K. Kielland, G.P. Kofinas, M.R. Turetsky, J. Yarie, A.H. Lloyd, and D.L. Taylor. 2010. Resilience of Alaska’s boreal forest to climatic change. Canadian Journal of Forest Research 40:1360-1370. https://doi.org/10.1139/X10-074
Ecological Stratification Working Group. 1995. A national ecological framework for Canada. Canadian Soil Information System (CANSIS). https://sis.agr.gc.ca/cansis/nsdb/ecostrat/index.html
Greene, D.F., and E.A. Johnson.1998. Seed mass and early survivorship of tree species in upland clearings and shelterwoods. Canadian Journal of Forest Research 28:1307-1316. https://doi.org/10.1139/X98-106
Hart S.J., J. Henkelman, P.D. McLoughlin, S.E. Nielsen, A. Truchon-Savard, and J.F. Johnstone. 2018. Examining forest resilience to changing fire frequency in a fire-prone region of boreal forest. Global Change Biology 25:869–884. https://doi.org/10.1111/gcb.14550
Johnstone, J.F., C.D. Allen, J.F Franklin, L.E. Frelich, B.J. Harvey, P.E. Higuera, M.C. Mack, R.K. Meentemeyer, M.R. Metz, G.L. Perry, and M.G.Turner. 2016. Changing disturbance regimes, ecological memory, and forest resilience. Frontiers in Ecology and the Environment 14:369–378. https://doi.org/10.1002/fee.1311
Johnstone, J.F., F.S. Chapin, T.N. Hollingsworth TN, M.C. Mack, V. Romanovsky, and M. Turetsky. 2010. Fire, climate change, and forest resilience in interior Alaska. Canadian Journal of Forest Research, 40(7), 1302–1312. https://doi.org/10.1139/X10-061
Kasischke, E.S., D.L. Verbyla, T.S. Rupp, A.D. McGuire, K.A. Murphy, R. Jandt, J.L. Barnes E.E. Hoy, P.A. Duffy, M. Calef , and M.R. Turetsky. 2010. Alaska’s changing fire regime - implications for the vulnerability of its boreal forests. Canadian Journal of Forest Research 40:313-1324. https://doi.org/10.1139/X10-098
NWT Centre fo Geomatics. 2012. Fire History (online dataset). NWT Centre fo Geomatics; Yellowknife, Northwest Territories, Canada. Retrieved July 15, 2020. https://www.geomatics.gov.nt.ca/en/fire-history
Santín C.,S.H. Doerr, A. Merino, R. Bryant, and N.J. Loader. 2016. Forest floor chemical transformations in a boreal forest fire and their correlations with temperature and heating duration. Geoderma 264:71–80. https://doi.org/10.1016/j.geoderma.2015.09.021
Viereck, L.A., C.T. Dyrness, K.V. Cleve, and M.J. Foote. 1983. Vegetation, soils, and forest productivity in selected forest types in interior Alaska. Canadian Journal of Forest Research 13:703-720. https://doi.org/10.1139/x83-101
Walker, X.J., J.L. Baltzer, S.G. Cumming, N.J. Day, C. Ebert, S. Goetz, J.F. Johnstone, S. Potter, B.M. Rogers, E.A. Schuur, M.R. Turetsky, and M.C. Mack. 2019. Increasing wildfires threaten historic carbon sink of boreal forest soils. Nature 572:520–523. https://doi.org/10.1038/s41586-019-1474-y
Walker, X.J., J.L. Baltzer, S.G. Cumming, N.J. Day, J.F. Johnstone, B.M. Rogers, K.Solvik, M.R. Turetsky, and M.C. Mack. 2018a. Soil organic layer combustion in boreal black spruce and jack pine stands of the Northwest Territories, Canada. International Journal of Wildland Fire 27:125–134. https://doi.org/10.1071/WF17095
Walker, X.J., B.M. Rogers, J.L. Baltzer, S.G. Cumming, N.J. Day, S. Goetz, J.F. Johnstone, E.A.G. Schuur, M.R. Turetsky, and M.C. Mack. 2018b. Cross-scale controls on carbon emissions from boreal forest megafires. Global Change Biology 24:4251–4265. https://doi.org/10.1111/gcb.14287
Walker, X.J., M.C. Mack, and J.F. Johnstone. 2017. Predicting Ecosystem Resilience to Fire from Tree Ring Analysis in Black Spruce Forests. Ecosystems 20:1137–1150. https://doi.org/10.1007/s10021-016-0097-5
Whitman, E., M.A. Parisien, D.K. Thompson, and M.D. Flannigan. 2019. Short-interval wildfire and drought overwhelm boreal forest resilience. Scientific Report. 9:1–12. https://doi.org/10.1038/s41598-019-55036-7