Documentation Revision Date: 2024-09-07
Dataset Version: 1
Summary
There is one file in comma separated values (.csv) format with this dataset.
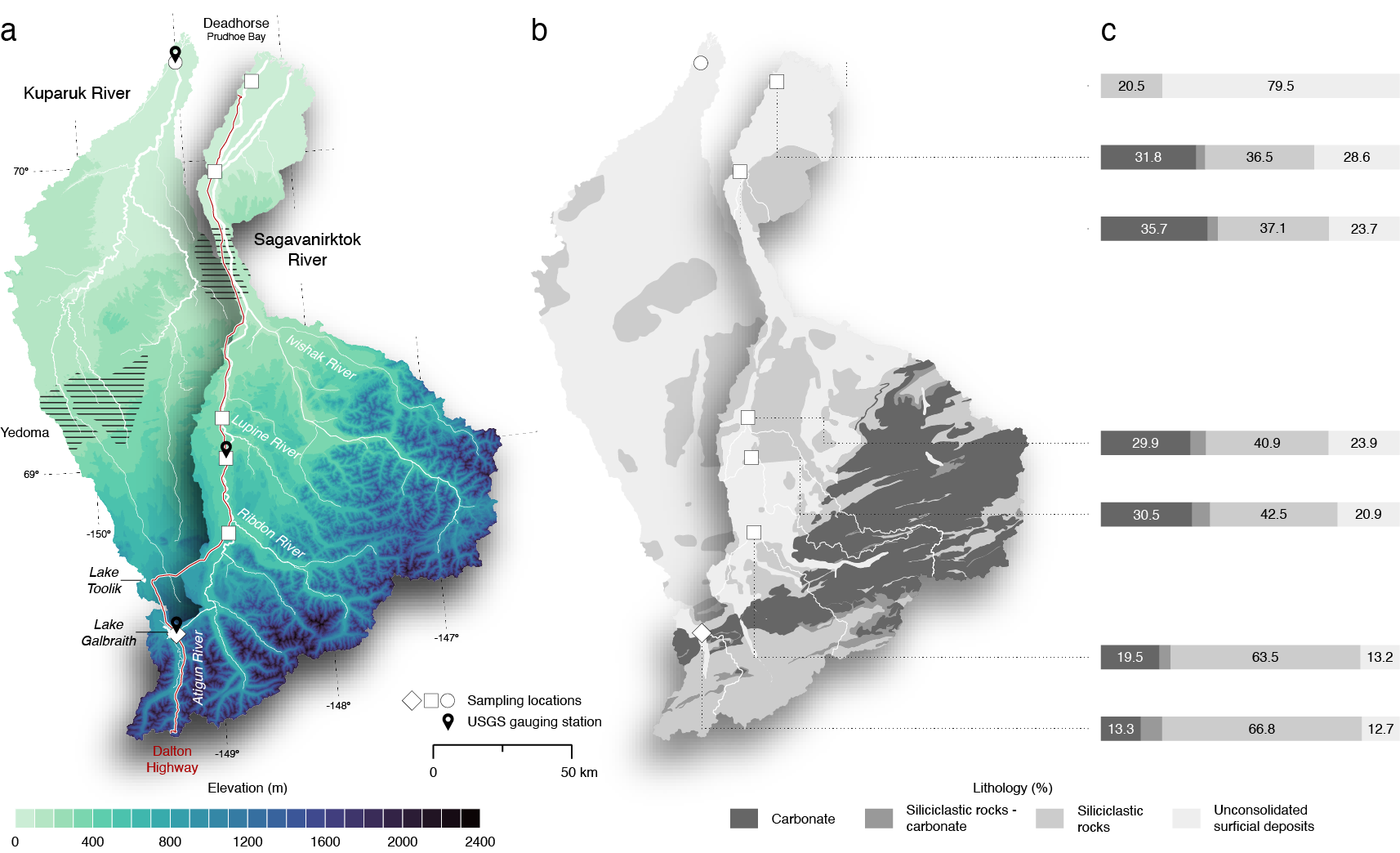
Figure 1. Topographical and lithological overview of the Sagavanirktok and Kuparuk River Basins: This figure illustrates (a) elevation, (b) underlying lithology based on Wilson et al. (2015) and (c) lithology percentages per sub-watershed. Sampling locations are marked as follows: a diamond for Atigun River, squares for Sagavanirktok River, and a circle for Kuparuk River.
Citation
Schwab, M.S., C. Elder, X. Xu, C.I. Czimczik, and C.E. Miller. 2024. ABoVE: Seasonal Dissolved Gases and Isotopes in Arctic Alaska Rivers. ORNL DAAC, Oak Ridge, Tennessee, USA. https://doi.org/10.3334/ORNLDAAC/2360
Table of Contents
- Dataset Overview
- Data Characteristics
- Application and Derivation
- Quality Assessment
- Data Acquisition, Materials, and Methods
- Data Access
- References
Dataset Overview
This dataset provides dissolved carbon dioxide (CO2) and methane (CH4) concentrations alongside their stable and radiocarbon isotopic compositions within the Arctic Sagavanirktok and Kuparuk River watersheds located on the North Slope of Alaska. The data were collected during the spring, fall, and summer seasons in 2022. In field separation of the bulk gaseous components (N2, CO2, and CH4) from the liquid phase was achieved using a degassing membrane contactor. Laboratory isotopic analyses were conducted at the W. M. Keck Carbon Cycle Accelerator Mass Spectrometer facility at UC Irvine. This collection aims to provide insights into the seasonal dynamics of greenhouse gas emissions in these critical Arctic environments, thereby contributing valuable information for climate change research and monitoring programs. The data are provided in comma separated values (CSV) format.
Project: Arctic-Boreal Vulnerability Experiment
The Arctic-Boreal Vulnerability Experiment (ABoVE) is a NASA Terrestrial Ecology Program field campaign being conducted in Alaska and western Canada, for 8 to 10 years, starting in 2015. Research for ABoVE links field-based, process-level studies with geospatial data products derived from airborne and satellite sensors, providing a foundation for improving the analysis, and modeling capabilities needed to understand and predict ecosystem responses to, and societal implications of, climate change in the Arctic and Boreal regions.
Related Publication
Schwab, M.S., C.D. Elder, X. Xu, C.I. Czimczik, and C.E. Miller. 2024. Isotopic Seasonality of Fluvial-Derived Greenhouse Gases Imply Active Layer Deepening. In review in Environmental Research Letters.
Acknowledgments
The research was carried out at the Jet Propulsion Laboratory, California Institute of Technology, under a contract with the National Aeronautics and Space Administration (80NM0018D0004). M.S.S. was supported by an appointment to the NASA Postdoctoral Program at the Jet Propulsion Laboratory, administered by Oak Ridge Associated Universities under contract with NASA.
Data Characteristics
Spatial Coverage: Sagavanirktok and Kuparuk Rivers, North Slope Borough, Alaska, USA.
ABoVE Reference Locations
Domain: Core
State/Territory: Alaska
Grid Cells: Ah001v000, Bh008v002, Bh008v003, Ch048v021, Ch049v021, Ch050v020, Ch052v018, Ch053v016, Ch053v017
Spatial Resolution: Point samples
Temporal Coverage: Spring: 2022-06-05 to 2022-06-09; Summer: 2022-07-15 to 2022-07-19; Fall: 2022-08-30 to 2022-09-04
Temporal Resolution: Samples were collected over the course of a week during spring and replicated for summer and fall.
Site Boundaries: Latitude and longitude are given in decimal degrees.
Site | Westernmost Longitude | Easternmost Longitude | Northernmost Latitude | Southernmost Latitude |
---|---|---|---|---|
Alaska | -149.3744 | -148.3033 | 70.3307 | 68.4519 |
Data File Information
There is one file with this dataset: Dissolved_GreenhouseGases_Sagavanirktok_Kuparuk_Rivers.csv.
Table 1. Variables in the data file
Variable | Units/Format | Description |
---|---|---|
id | - | Unique identifier for each discrete measurement |
location | - | Name of the nearest location to the measurement site |
river | - | River name corresponding to water collection site |
latitude | degrees north | Latitude coordinate of the sampling site, expressed in decimal degrees |
longitude | degrees east | Longitude coordinate of the sampling site, expressed in decimal degrees |
date | YYYY-MM-DD | Date of sample collection |
season | - | Season corresponding to the time of sample collection |
discharge | m3 s-1 | Discharge measured at the USGS gauging station 15905100, 15908000, and 15896000 in cubic meters per second |
co2 | µmol L-1 | Dissolved CO2 concentration, expressed in micromoles per liter |
co2_f14c | 1 | Fraction modern of dissolved CO2 (CO2-F14C) |
co2_f14c_sd | 1 | Standard deviation corresponding to the fraction modern of dissolved CO2 |
co2_d13c | ‰ | Stable carbon isotopic composition of dissolved CO2 (CO2-d13C) in per mille (‰) |
co2_d13c_sd | ‰ | Standard deviation corresponding to the stable isotopic composition of dissolved CO2 in per mille (‰) |
ch4 | µmol L-1 | Dissolved CH4 concentration, expressed in micromoles per liter (µmol L-1) |
ch4_f14c | 1 | Fraction modern of dissolved CH4 (CH4-F14C) |
ch4_f14c_sd | 1 | Standard deviation corresponding to the fraction modern of dissolved CH4 |
ch4_d13c | ‰ | Stable carbon isotopic composition of dissolved CH4 (CH4-d13C) in per mille (‰) |
ch4_d13c_sd | ‰ | Standard deviation corresponding to the stable isotopic composition of dissolved CH4 in per mille (‰) |
Application and Derivation
This study represents the first collection of dissolved CO2 and CH4 stable (δ13C) and radiocarbon (14C) isotopic data from two Arctic river systems during the ice-free period. By examining temporal and geomorphological gradients, patterns and pinpoint source contributions of these greenhouse gases can be discserned to enhance understanding of Arctic fluvial biogeochemistry.
Quality Assessment
The measurement uncertainties and quality controls for δ13C and F14C data in the provided study were addressed using high-precision instruments and robust calibration methods. For F14C, uncertainties were mitigated through corrections for process blank contamination based on the methods detailed by Elder (2018) and Elder et al. (2018). The 14C content was measured using an accelerator mass spectrometer, with procedural blanks included to account for any background contamination. For δ13C, the data quality was ensured by using isotope-ratio mass spectrometry calibrated against known laboratory standards of CH4 and CO2. This calibration helps in maintaining the accuracy and consistency of δ13C measurements across different samples. The procedures used effectively minimize biases and enhance the reliability of the carbon isotope data reported.
Data Acquisition, Materials, and Methods
Sample Collection
Dissolved CO2 and CH4 were sampled from six locations along the Sagavanirktok River, spanning a geomorphological gradient, and from a single site in the Kuparuk River delta. These collections occurred shortly after ice breakup (5-9 June), during summer (15-19 July), and in fall (30 August to 3 September) of 2022. A Liqui-Cel membrane contactor was used (Membrana, 3M Company) to extract the dissolved gases as described in Elder et al. (2018, 2019). River water was drawn through tubing connected to a peristaltic pump from the riverbank, maintaining an average flow rate of 1.3 L min-1 for durations ranging from 90 to 180 min. The setup included a filtration system with two stainless steel mesh filters (178 μm (T-29595-39) and 40 μm (T-2959-35), Cole-Parmer) and two polypropylene sediment depth filters (20 μm SD-25-0520 Flow-Pro and 5 μm SD-25-0505 Hydronix, FreshWatersystems) to remove suspended particulates. The separation of the bulk gaseous components (N2, CO2, and CH4) from the liquid phase was achieved using a degassing membrane contactor. These gases were then captured in a pre-evacuated, pre-weighed 2 L stainless steel canister equipped with a stainless-steel bellows sealed valve (SS-4BG, Swagelok). To mitigate the risk of cross-contamination, all filters were replaced, and the system was flushed with river water for 30 min before each sampling session.
Laboratory Analyses
All isotopic analyses were conducted at the W. M. Keck Carbon Cycle Accelerator Mass Spectrometer facility at UC Irvine. Canisters were weighed to quantify the mass of accumulated dissolved gases before connecting them to a flow-through vacuum extraction line designed for the sequential separation and purification of CO2 and CH4 (Pack et al., 2015). Upon introducing the sample gas into the vacuum line, CO2 was cryogenically isolated from CH4, carbon monoxide (CO), and non-condensable gases (e.g., N2) using a liquid nitrogen trap. CO was converted into CO2 at 290ºC with cupric oxide and captured in another liquid nitrogen trap. Similarly, CH4 was oxidized to CO2 in a 975ºC furnace in the presence of cupric oxide. CH4-derived CO2 and sample CO2 were further purified on the vacuum line, quantified manometrically, and chemically reduced into graphite using the sealed-tube zinc-reduction method (Xu et al., 2007). Isotopic standards were extracted using the same methods to evaluate the procedural contamination of the sampling methods/extraction line system.
The 14C content of the graphitized samples was measured with a 0.5 MV 1.5SDH-2 accelerator mass spectrometer (AMS, National Electrostatics Corporation) alongside procedural blanks and analytical standards (Beverly et al., 2010). To compensate for process blank contamination, all samples were corrected following the methods described in Elder (2018) and Elder et al. (2018) and reported as fraction modern, F14C (Reimer et al., 2004). For samples > 0.2 mg C, an aliquot was analyzed for δ13C using isotope-ratio mass spectrometry (IRMS) (GasBench II coupled to a Finnigan Delta Plus IRMS (Thermo Scientific)). Stable isotope samples were calibrated against gaseous CH4 and CO2 laboratory standards and reported in δ13C notation relative to Vienna Pee Dee Belemnite (VPDB).
The CO2 and CH4 concentrations were computed from the extracted yields and corrected to reflect a 90% efficiency of the vacuum line extraction (Elder 2018). Subsequently, these concentrations were refined by applying the efficiencies of the Liqui-Cel extraction for CO2 and CH4, which were empirically determined based on the average flow rate (x) as follows:
CO2 efficiency = -0.4 * ln(x) + 4 (1)
CH4 efficiency = -0.49 * x2 + 1.1 * x + 0.4. (2)
Data Access
These data are available through the Oak Ridge National Laboratory (ORNL) Distributed Active Archive Center (DAAC).
ABoVE: Seasonal Dissolved Gases and Isotopes in Arctic Alaska Rivers
Contact for Data Center Access Information:
- E-mail: uso@daac.ornl.gov
- Telephone: +1 (865) 241-3952
References
Beverly, R.K., W. Beaumont, D. Tauz, K.M. Ormsby, K.F. von Reden, G.M. Santos, and J.R. Southon. 2010.The Keck Carbon Cycle AMS Laboratory, University of California, Irvine: Status Report. Radiocarbon 52:301–309. https://doi.org/10.1017/S0033822200045343
Elder, C.D. 2018. Understanding Methane and Carbon Dioxide Emissions from Climate-Sensitive Northern Lakes (Doctoral Dissertation). University of California, Irvine. https://escholarship.org/uc/item/7hz363ps
Elder, C.D., X. Xu, J. Walker, J.L. Schnell, K.M. Hinkel, A. Townsend-Small, C.D. Arp, J.W. Pohlman, B.V. Gaglioti, and C.I. Czimczik. 2018. Greenhouse gas emissions from diverse Arctic Alaskan lakes are dominated by young carbon. Nature Climate Change 8:166–171. https://doi.org/10.1038/s41558-017-0066-9
Elder, C.D., M. Schweiger, B. Lam, E.D. Crook, X. Xu, J. Walker, K.M.Walter Anthony, and C.I. Czimczik. 2019. Seasonal Sources of Whole-Lake CH4 and CO2 Emissions from Interior Alaskan Thermokarst Lakes. Journal of Geophysical Research: Biogeosciences 124:1209–1229. https://doi.org/10.1029/2018JG004735
Pack, M., X. Xu, M. Lupascu, J.D. Kessler, and C.I. Czimczik. 2015. A rapid method for preparing low volume CH4 and CO2 gas samples for 14C AMS analysis. Organic Geochemistry 78:89–98. https://doi.org/10.1016/j.orggeochem.2014.10.010
Reimer, P.J., T.A. Brown, and R.W. Reimer. 2004. Discussion: Reporting and Calibration of Post-Bomb 14C Data. Radiocarbon 46:1290–1304. https://doi.org/10.1017/S0033822200033154
Schwab, M.S., C.D. Elder, X. Xu, C.I. Czimczik, and C.E. Miller. 2024. Isotopic Seasonality of Fluvial-Derived Greenhouse Gases Imply Active Layer Deepening. In review in Environmental Research Letters.
Wilson, F.H., C.P. Hults, C.P. Mull, and S.M. Karl. 2015. Geologic map of Alaska: U.S. Geological Survey, Scientific Investigations Map 3340, pamphlet 196. https://doi.org/10.3133/sim3340
Xu, X., S.E. Trumbore, S. Zheng, J.R. Southon, K.E. McDuffee, M. Luttgen, and J. Liu. 2007. Modifying a sealed tube zinc reduction method for preparation of AMS graphite targets: Reducing background and attaining high precision. Nuclear Instruments and Methods in Physics Research, Section B: Beam Interactions with Materials and Atoms 259:320–329. https://doi.org/10.1016/j.nimb.2007.01.175