Documentation Revision Date: 2019-04-18
Dataset Version: 1
Summary
A half-degree resolution mask was created of the ABoVE domain and used to clip from the global TRENDY and North America (NACP regional) model outputs. The mask was transformed to match the different native resolutions of the 20 models as needed. Mean annual maps were produced for the reference year 2003 for NEE, GPP, Rh, Ra, NPP, and C soil by averaging the available monthly model output and preserving the native spatial resolution for each model. Maps were produced for the multi-model standard deviation (σ) from the individual mean annual maps.
There are six data files in GeoTIFF (.tif) format with this dataset.
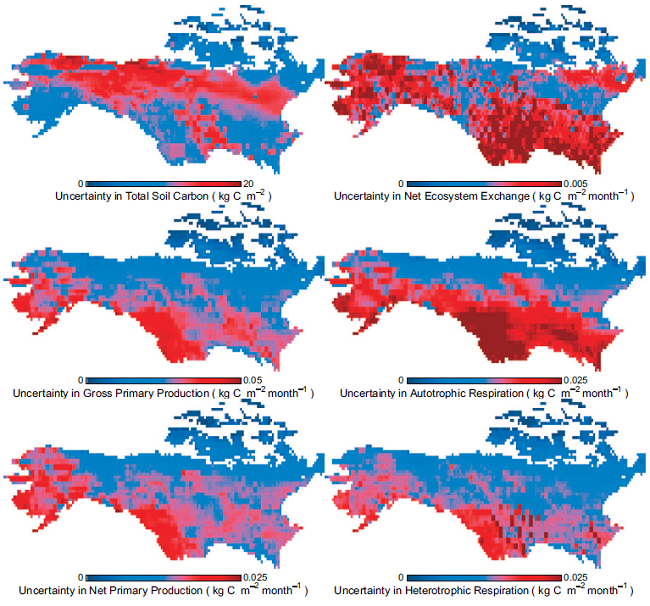
Figure 1. Uncertainty in ecosystem carbon stocks and fluxes across the ABoVE domain. Flux units are in kg C/m2/month; carbon stock units are in kg C/m2. Image from Fisher et al. (2018).
Citation
Fisher, J.B. 2019. ABoVE: Multi-model Uncertainty of Carbon Stocks and Fluxes across ABoVE Domain, 2003. ORNL DAAC, Oak Ridge, Tennessee, USA. https://doi.org/10.3334/ORNLDAAC/1652
Table of Contents
- Dataset Overview
- Data Characteristics
- Application and Derivation
- Quality Assessment
- Data Acquisition, Materials, and Methods
- Data Access
- References
Dataset Overview
This dataset provides estimates of the uncertainty in components of the carbon cycle including: soil carbon stock, autotrophic respiration (Ra), heterotrophic respiration (Rh), net ecosystem exchange (NEE), net primary production (NPP), and gross primary productivity (GPP) across the entire ABoVE Study Domain at 0.5-degree resolution for the reference year 2003. The uncertainties were calculated from the multi-model (n = 20) disagreement, i.e. standard deviation, from the Trends in Net Land Atmosphere Carbon Exchanges program (TRENDY) and the North American Carbon Program (NACP) regional synthesis model outputs averaged to annual means. This total uncertainty integrates both structural uncertainty of land-surface physics among models as well as inherent parametric uncertainty introduced within models, and uncertainty from forcing data.
A half-degree resolution mask was created of the ABoVE domain and used to clip from the global TRENDY and North America (NACP regional) model outputs. The mask was transformed to match the different native resolutions of the 20 models as needed. Mean annual maps were produced for the reference year 2003 for NEE, GPP, Rh, Ra, NPP, and C soil by averaging the available monthly model output and preserving the native spatial resolution for each model. Maps were produced for the multi-model standard deviation (σ) from the individual mean annual maps.
Project: Arctic-Boreal Vulnerability Experiment
The Arctic-Boreal Vulnerability Experiment (ABoVE) is a NASA Terrestrial Ecology Program field campaign based in Alaska and western Canada between 2016 and 2021. Research for ABoVE links field-based, process-level studies with geospatial data products derived from airborne and satellite sensors, providing a foundation for improving the analysis and modeling capabilities needed to understand and predict ecosystem responses and societal implications.
Related Publications:
Fisher, J.B., Hayes, D.J., Schwalm, C.R., Huntzinger, D.N., Stofferahn, E., Schaefer, K., Luo, Y., Wullschleger, S.D., Goetz, S., Miller, C.E., Griffith, P., Chadburn, S., Chatterjee, A., Ciais, P., Douglas, T.A., Genet, H., Ito, A., Neigh, C.S.R., Poulter, B., Rogers, B.M., Sonnentag, O., Tian, H., Wang, W., Xue, Y., Yang, Z.-L., Zeng, N., 2018. Missing pieces to modeling the Arctic-Boreal puzzle. Environmental Research Letters 13(2): 020202. https://doi.org/10.1088/1748-9326/aa9d9a
Fisher, J. B., Sikka, M., Oechel, W. C., Huntzinger, D. N., Melton, J. R., Koven, C. D., Ahlström, A., Arain, M. A., Baker, I., Chen, J. M., Ciais, P., Davidson, C., Dietze, M., El-Masri, B., Hayes, D., Huntingford, C., Jain, A. K., Levy, P. E., Lomas, M. R., Poulter, B., Price, D., Sahoo, A. K., Schaefer, K., Tian, H., Tomelleri, E., Verbeeck, H., Viovy, N., Wania, R., Zeng, N., and Miller, C. E. 2014. Carbon cycle uncertainty in the Alaskan Arctic, Biogeosciences, 11, 4271-4288, https://doi.org/10.5194/bg-11-4271-2014
Acknowledgements:
This work was supported by NASA’s Arctic Boreal Vulnerability Experiment (ABoVE), grant number NNN13D504T.
Data Characteristics
Spatial Coverage: ABoVE Study Domain, covering all of Alaska and all provinces of Canada
ABoVE Reference Locations:
Domain: Entire ABoVE Study Domain
State/territory: Alaska and Canada
Grid cell(s): All “A” grid cells
Spatial Resolution: 0.5 degree
Temporal Coverage: 2003-01-01 to 2003-12-31
Temporal Resolution: One time (reference year 2003)
Study Area (All latitude and longitude given in decimal degrees)
Site |
Westernmost Longitude |
Easternmost Longitude |
Northernmost Latitude |
Southernmost Latitude |
---|---|---|---|---|
ABoVE Study Domain |
- 176.124747 |
-67.124747 |
81.409411 |
39.409411 |
Data File Information:
There are six data files in GeoTIFF (.tif) format. The data are uncertainties in ecosystem carbon stocks and fluxes across the ABoVE domain at 0.5-degree resolution calculated from multi-model disagreement, i.e. standard deviations.
Table 1. File names and descriptions
File name | Data Units | Description |
---|---|---|
autotrophic_respiration_std_dev.tif | kg/m2/month | The standard deviation for autotrophic respiration (Ra) |
gross_primary_production_std_dev.tif | kg/m2/month | The standard deviation for gross primary production (GPP) |
heterotrophic_respiration_std_dev.tif | kg/m2/month | The standard deviation for heterotrophic respiration (Ha) |
net_primary_production_std_dev.tif | kg/m2/month | The standard deviation for net primary production (NPP) |
total_soil_carbon_std_dev.tif | kg/m2 | The standard deviation for total soil carbon content |
net_ecosystem_exchange_std_dev.tif | kg/m2/month | The standard deviation for net ecosystem exchange (NEE) |
Spatial Properties: Data bands in each file: 1. No data value: -9999, Resolution: 0.5 degree, EPSG: 4326
Application and Derivation
Our analysis of site representation showed critical areas are not well represented. This analysis will help inform decisions for upcoming field campaigns in the region. These results are fundamental to future research in the Alaskan Arctic and boreal region to reduce uncertainties in the Arctic and boreal carbon cycle.
Quality Assessment
Low uncertainty regions may be classed as such due to the uncertainty definition in the study, but models may have converged due to equifinality or other shared assumptions, while uncertainty by other definitions may be large. See Fisher et al. (2018) for more discussion.
Data Acquisition, Materials, and Methods
Model output from the most recent versions of NACP regional synthesis models (Huntzinger et al., 2012) and TRENDY models were used to define carbon cycle uncertainties in the Arctic Boreal Vulnerability Experiment (ABoVE) domain. This total uncertainty integrates both structural uncertainty of land-surface physics among models as well as inherent parametric uncertainty introduced within models, and uncertainty from forcing data. Variables assessed included NEE, gross primary production (GPP), heterotrophic respiration (Rh), autotrophic respiration (Ra), net primary production (NPP), and soil carbon stock (Csoil). Some models provided GPP and NPP, but not Ra, while others provided GPP and Ra, but not NPP, so the missing term in those equations with one unknown.
Table 2: Models and variables in the uncertainty study. The model references are included in the Reference section below..
Model Short Name | Variables Assessed | Model Intercomparison Project (NACP and TRENDY) | Reference |
---|---|---|---|
BEPS | GPP, NPP, Rh, Ra | NACP | Chen et al. (1999) |
CanIBIS | GPP, NPP, Rh, Ra, soil C | NACP | El Maayar et al. (2002) |
CASA-GFED | GPP, Rh | NACP | van der Werf et al. (2004 |
CASA-TRANSCOM | GPP, NPP, Rh, Ra, soilC | NACP | Randerson et al. (1997) |
CLM-CASA | GPP, NPP, Rh, Ra, soil C | NACP | Randerson et al. (2009) |
CLM4-CN | GPP, NPP, Rh, Ra, soil C | NACP & TRENDY | Thornton et al. (2007) |
DLEM | GPP, NPP, Ra, Rh, SoilC | NACP | Tian et al. (2010) |
HYLAND | GPP, NPP, Rh, Ra, soil C | TRENDY | Levy et al. (2004) |
ISAM | GPP, NPP, Rh, Ra, soilC | NACP | Jain and Yang (2005) |
LPJ-wsl | GPP, NPP, Rh, Ra, soil C | NACP & TRENDY | Sitch et al. (2003) |
LPJ-GUESS | GPP, NPP, Rh, Ra, soil C | TRENDY | Smith et al. (2001) |
MOD17+ | GPP, NPP, Rh, Ra, soilC | NACP | Zhao et al. (2005) |
OCN | GPP, NPP, Rh, Ra, soil C | TRENDY | Zaehle et al. (2010) |
ORCHIDEE | GPP, NPP, Rh, Ra, soil C | NACP & TRENDY | Krinner et al. (2005) |
SDGVM | GPP, NPP, Rh, Ra, soil C | TRENDY | Cramer et al. (2001) |
SIB3 | GPP, NPP, Rh, Ra | NACP | Baker et al. (2008) |
TEM6 | GPP, NPP, Rh, Ra, soil C | NACP | Hayes et al. (2011) |
TRIFFID | GPP, NPP, Rh, Ra, soil C | TRENDY | Clark et al. (2011) |
VEGAS | GPP, NPP, Rh, Ra, soil C | TRENDY | Zeng et al. (2005) |
VEGAS2 | GPP, NPP, Rh, Ra | NACP | Zeng et al. (2005) |
Most variables were identical across NACP regional and TRENDY, with the exception of net CO2 flux, reported as net biome production (NBP) for TRENDY (and net ecosystem production, NEP, for HYLAND only), but oppositely reported as NEE for NACP regional. To equate the CO2 flux between both MIPs, the sign for TRENDY was reversed (and converted time units of seconds to months), though technically NBP should include additional fluxes from fire and other disturbances as well as lateral carbon transport that NEE would not include. Models LPJwsl and VEGAS from TRENDY were not converted because their values were already in the units of NACP. Models HYLAND and SDGVM in TRENDY reported net CO2 flux values in the incorrect sign so the sign was reversed.
Model Output Processing
A half-degree resolution mask was created of the ABoVE domain used to clip from the global (TRENDY) and North America (NACP regional) model output. The masks were transformed to match the different native resolutions of the models. Mean annual maps were produced for the reference year 2003 for NEE, GPP, Rh, Ra, NPP, and C soil by averaging the available monthly model output and preserving the native spatial resolution for each model.
Maps were produced for the multi-model standard deviation (σ) from the individual mean annual maps. All models were arithmetically downscaled with coarser resolutions to 0.5 degree. Pixels that overlapped with one another across models were used to calculate the individual half-degree pixel averages (Fisher et al., 2014).
Data Access
These data are available through the Oak Ridge National Laboratory (ORNL) Distributed Active Archive Center (DAAC).
ABoVE: Multi-model Uncertainty of Carbon Stocks and Fluxes across ABoVE Domain, 2003
Contact for Data Center Access Information:
- E-mail: uso@daac.ornl.gov
- Telephone: +1 (865) 241-3952
References
Baker, I. T., L. Prihodko, A.S. Denning, M. Goulden, S. Miller, and H.R. da Rocha. Seasonal drought stress in the Amazon: Reconciling models and observations. 2008. J. Geophys. Res., 113. https://doi.org/10.1029/2007jg000644
Chen, J. M., Liu, J., Cihlar, J., and Goulden, M. L. 1999. Daily canopy photosynthesis model through temporal and spatial scaling for remote sensing applications, Ecol. Modell., 124, 99–119. https://doi.org/10.1016/S0304-3800(99)00156-8
Clark, D. B., Mercado, L. M., Sitch, S., Jones, C. D., Gedney, N., Best, M. J., Pryor, M., Rooney, G. G., Essery, R. L. H., Blyth, E., Boucher, O., Harding, R. J., Huntingford, C., and Cox, P. M. 2011. The Joint UK Land Environment Simulator (JULES), model description – Part 2: Carbon Fluxes and vegetation dynamics. Geosci. Model Dev., 4, 701–722. https://doi.org/10.5194/gmd-4-701-2011
Cramer, W., Bondeau, A., Woodward, F. I., Prentice, I. C., Betts, R. A., Brovkin, V., Cox, P. M., Fisher, V., Foley, J. A., Friend, A. D., Kucharik, C., Lomas, M. R., Ramankutty, N., Sitch, S., Smith, B., White, A., and Young-Molling, C. 2001. Global response of terrestrial ecosystem structure and function to CO2 and climate change: results from six dynamic global vegetation models, Glob. Change Biol., 7, 357–373. https://doi.org/10.1046/j.1365-2486.2001.00383.x
El Maayar, M., Price, D. T., Black, T. A., Humphreys, E. R., and Jork, E. M. 2002. Sensitivity tests of the integrated biosphere simulator to soil and vegetation characteristics in a pacific coastal coniferous forest. Atmos.-Oc., 40, 313–332. https://doi.org/10.3137/ao.400303
Fisher, J.B., Hayes, D.J., Schwalm, C.R., Huntzinger, D.N., Stofferahn, E., Schaefer, K., Luo, Y., Wullschleger, S.D., Goetz, S., Miller, C.E., Griffith, P., Chadburn, S., Chatterjee, A., Ciais, P., Douglas, T.A., Genet, H., Ito, A., Neigh, C.S.R., Poulter, B., Rogers, B.M., Sonnentag, O., Tian, H., Wang, W., Xue, Y., Yang, Z.-L., Zeng, N., 2018. Missing pieces to modeling the Arctic-Boreal puzzle. Environmental Research Letters 13(2): 020202. https://doi.org/10.1088/1748-9326/aa9d9a
Fisher, J. B., Sikka, M., Oechel, W. C., Huntzinger, D. N., Melton, J. R., Koven, C. D., Ahlström, A., Arain, M. A., Baker, I., Chen, J. M., Ciais, P., Davidson, C., Dietze, M., El-Masri, B., Hayes, D., Huntingford, C., Jain, A. K., Levy, P. E., Lomas, M. R., Poulter, B., Price, D., Sahoo, A. K., Schaefer, K., Tian, H., Tomelleri, E., Verbeeck, H., Viovy, N., Wania, R., Zeng, N., and Miller, C. E. 2014. Carbon cycle uncertainty in the Alaskan Arctic. Biogeosciences, 11, 4271-4288. https://doi.org/10.5194/bg-11-4271-2014
Hayes, D. J., McGuire, A. D., Kicklighter, D. W., Gurney, K. R., Burnside, T. J., and Melillo, J. M. 2011. Is the northern high-latitude land-based CO2 sink weakening? Global Biogeochem. Cy., 25. https://doi.org/10.1029/2010GB003813.
Huntzinger, D. N., Post, W. M., Wei, Y., Michalak, A. M., West, T. O., Jacobson, A. R., Baker, I. T., Chen, J. M., Davis, K. J., Hayes, D. J., Hoffman, F. M., Jain, A. K., Liu, S., McGuire, A. D., Neilson, R. P., Potter, C., Poulter, B., Price, D., Raczka, B. M., Tian, H. Q., Thornton, P., Tomelleri, E., Viovy, N., Xiao, J., Yuan, W., Zeng, N., Zhao, M., and Cook, R. 2012. North American Carbon Program (NACP) regional interim synthesis: terrestrial biospheric model intercomparison. Ecol. Modell., 232, 144–157. https://doi.org/10.1016/j.ecolmodel.2012.02.004
Jain, A. K. and Yang, X. 2005. Modeling the effects of two different land cover change data sets on the carbon stocks of plants and soils in concert with CO2 and climate change, Global Biogeochem. Cy., 19. https://doi.org/10.1029/2004gb002349
Krinner, G., N. Viovy, N. de Noblet, J. Ogee, J. Polcher, P. Friedlingstein, P. Ciais, S. Sitch, and I.C. Pierce. 2005. A dynamic global vegetation model for studies of the coupled atmosphere-biosphere system. https://doi.org/10.1029/2003GB002199
Levy, P. E., M.G.R. Cannell, and A.D. Friend. 2004. Modelling the impact of future changes in climate, CO2 concentration and land use on natural ecosystems and the terrestrial carbon sink. Glob. Environ. Change, 14, Issue 1, 21–30. https://doi.org/10.1016/j.gloenvcha.2003.10.005
Randerson, J. T., Hoffman, F. M., Thornton, P. E., Mahowald, N. M., Lindsay, K., Lee, Y.-H., Nevison, C. D., Doney, S. C., Bonan, G., Stöckli, R., Covey, C., Running, S. W., and Fung, I. Y. 2009. Systematic assessment of terrestrial biogeochemistry in coupled climate-carbon models. Glob. Change Biol., 15, 2462–2484. https://doi.org/10.1111/j.1365-2486.2009.01912.x
Randerson, J. T., Thompson, M. V., Conway, T. J., Fung, I. Y., and Field, C. B. 1997. The contribution of terrestrial sources and sinks to trends in the seasonal cycle of atmospheric carbon dioxide. Global Biogeochem. Cy., 11, 535–560. https://doi.org/10.1029/97GB02268
Sitch, S., B. Smith, I.C. Prentice, A. Arneth, A. Bondeau, W. Cramer, J.O. Kaplan, W. Lucht, M. T. Sykes, K. Thonicke, and S. Venevsky. 2003. Evaluation of ecosystem dynamics, plant geography and terrestrial carbon cycling in the LPJ dynamic global vegetation model. Global Biogeochem. Cy., (9), 2, 161-185. https://doi.org/10.1046/j.1365-2486.2003.00569.x
Smith, B., Prentice, I. C., and Sykes, M. T. 2001. Representation of vegetation dynamics in modelling of terrestrial ecosystems: comparing two contrasting approaches within European climate space. Glob. Ecol. Biogeogr., 10, 621–637. https://doi.org/10.1046/j.1466-822X.2001.t01-1-00256.x
Thornton, P. E., Lamarque, J.-F., Rosenbloom, N. A., and Mahowald, N. M. 2007. Influence of carbon-nitrogen cycle coupling on and model response to CO2 fertilization and climate variability. Global Biogeochem. Cy., 21,1-15. https://doi.org/10.1029/2006GB002868
Tian, H., Chen, G., Liu, M., Zhang, C., Sun, G., Lu, C., Xu, X., Ren, W., Pan, S., and Chappelka, A. 2010. Model estimates of net primary productivity, evapotranspiration, and water use efficiency in the terrestrial ecosystems of the southern United States during 1895– 2007. Forest Ecol. Manage., 259, 1311–1327. https://doi.org/10.1016/j.foreco.2009.10.009
van der Werf, G. R., Randerson, J. T., Collatz, G. J., Giglio, L., Kasibhatla, P. S., Arellano, A. F., Olsen, S. C., and Kasischke, E. S. 2004. Continental-scale partitioning of fire emissions during the 1997–2001 El Niño/La Niña period. Science, 303, 73–76. https://doi.org/10.1126/science.1090753
Zaehle, S., Friend, A. D., Friedlingstein, P., Dentener, F., Peylin, P., and Schulz, M. 2010. Carbon and nitrogen cycle dynamics in the O-CN land surface model, II: The role of the nitrogen cycle in the historical terrestrial C balance. Global Biogeochem. Cy., 24, 1–14. https://doi.org/10.1029/2009GB003522
Zeng, N., Qian, H., Roedenbeck, C., and Heimann, M. 2005. Impact of 1998-2002 midlatitude drought and warming on terrestrial ecosystem and the global carbon cycle. Geophys. Res. Lett., 32, L22709. https://doi.org/10.1029/2005gl024607
Zhao, M. S., Heinsch, F. A., Nemani, R. R., and Running, S. W. 2005. Improvements of the MODIS terrestrial gross and net primary production global data set. Remote Sens. Environ., 98, 164–176. https://doi.org/10.1016/j.rse.2004.12.011